The role of tomato wild relatives in breeding disease-free varieties
Abstract
Cultivated tomato (Solanum lycopersicum) is one of the most economically important and widely grown vegetable crops worldwide. However, tomato plants are often affected by biotic and abiotic stresses that reduce yield and affect fruit quality. Phenotypic diversity is evident in cultivated tomatoes, particularly for horticultural traits, but genetic diversity is rather narrow. Major disease resistance genes for different pathogens such as viruses, fungi, bacteria and nematodes are mainly derived from wild tomato species and introgressed into cultivated tomatoes. Here, we list the major disease and insect-pest resistance genes identified in S. pimpinellifolium, S. habrochaites, S. peruvianum, S. chilense, S. pennellii, S. galapagense, S. arcanum and S. neorickii with perspective on the gap between current knowledge on tomato wild relatives and the knowledge that is needed.
Keywords
Tomato, crop wild relatives, disease resistance, genomics, plant genetic resources, plant breeding
Tomato wild relatives
Crop wild relatives (CWRs) are potential sources of allelic variation useful to overcome biotic and abiotic stresses as they often grow and reproduce in marginal habitats (Bohra et al., 2021; Ortiz, 2015). Tomato wild relatives are native to South America, distributed from the coastal region of Ecuador to northern Chile, including the Galápagos Islands (Darwin, Knapp, & Peralta, 2003). These regions have extremely varied environments within short distances due to differences in altitude (Andean geography) and diverse ecological habitats, which led to local adaptation and generation of large genetic diversity among (Figure 1) and within wild tomato species. Solanum section Lycopersicon (Mill.) Wettst. consists of cultivated tomato (S. lycopersicum) and 12 wild relatives (Ramírez-Ojeda et al., 2021). The Tomato Genetics Resource Center (C.M. Rick TGRC, University of California-Davis, USA, https://tgrc.ucdavis.edu/) hosts the largest genetic stocks of wild tomato collections, with over 900 accessions. The largest collection belongs to accessions of S. pimpinellifolium (~300) followed by S. habrochaites (~120), S. peruvianum (~100), S. chilense (~100), and S. pennellii (~50), respectively (Table 1). The World Vegetable Center (WorldVeg, Taiwan https://genebank.worldveg.org/#/) and the United States Department of Agriculture, Agricultural Research Service (USDA-ARS, https://www.ars-grin.gov/) genebanks also maintain the second and third largest wild tomato collections, respectively (Table 1). However, the majority of their wild tomato collection was originally obtained from the TGRC collection. Furthermore, these genebanks harbour an extensive collection of introgression lines derived from different tomato wild species (Ebert & Schafleitner, 2015).
Species |
No. of accessions per genebank |
||
|
TGRC |
WorldVeg |
USDA-ARS |
S. pimpinellifolium |
290 |
342 |
338 |
S. habrochaites |
120 |
139 |
49 |
S. peruvianum |
69 |
116 |
11 |
S. chilense |
115 |
46 |
1 |
S. pennellii |
47 |
59 |
5 |
S. corneliomulleri |
53 |
10 |
12 |
S. neorickii |
47 |
12 |
8 |
S. galapagense |
28 |
27 |
4 |
S. arcanum |
45 |
4 |
3 |
S. cheesmaniae |
12 |
17 |
5 |
S. chmielewskii |
16 |
11 |
0 |
S. lycopersicoides |
23 |
5 |
0 |
S. sitiens |
13 |
5 |
2 |
S. huaylasense |
16 |
0 |
0 |
S. ochranthum |
7 |
0 |
2 |
S. juglandifolium |
5 |
1 |
0 |
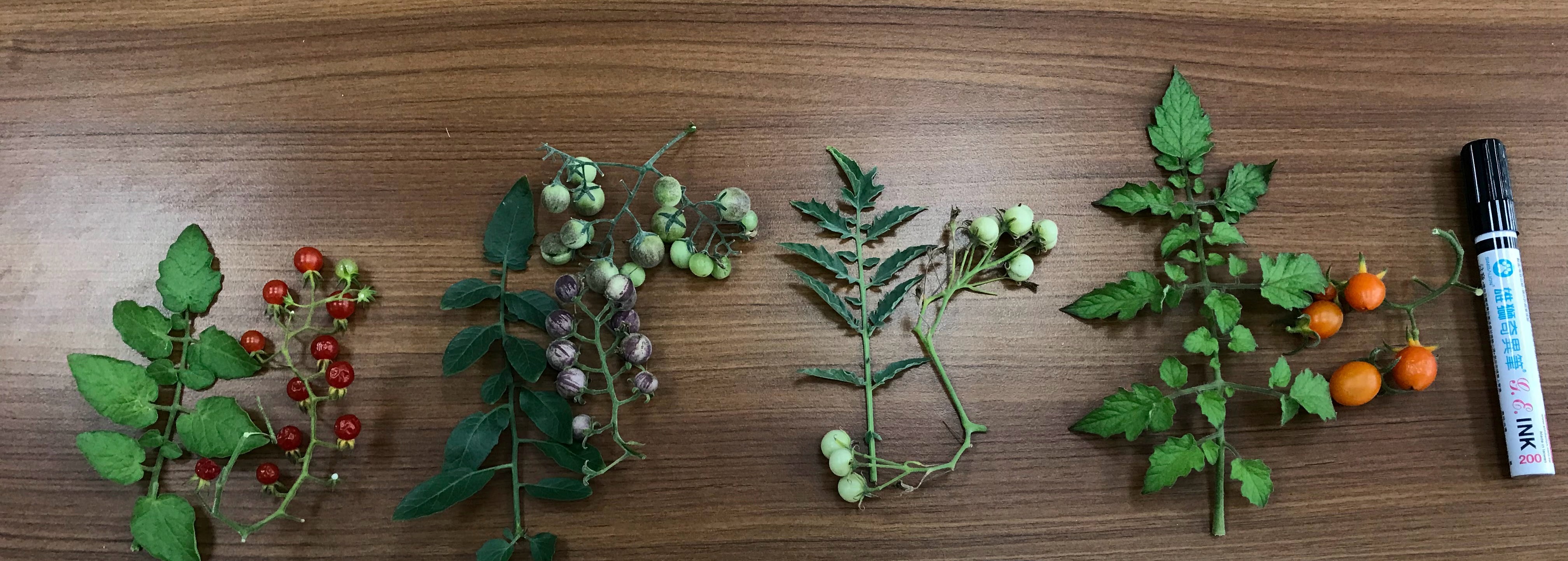
Utilization of tomato wild relatives for biotic stress breeding
Domestication has increased the phenotypic diversity of cultivated tomatoes but may have narrowed their resistance to biotic and abiotic constraints as selection ensued (Vu, Das, Tran, Hong, & Kim, 2020). Tomato wild relatives germplasm harbour natural resistance to various diseases and insect pests. Sources of genetic resistance to many of the biotic stresses faced by cultivated tomatoes have been identified in some accessions of wild tomato species that have been historically used to introduce resistance (R) genes into cultivated tomato varieties. It should be noticed that there is genetic diversity for biotic resistance response within tomato wild species, and only a few accessions within each species have been identified as sources of resistance ( (Ebert et al., 2015) and references). We list major R genes found in and introgressed from wild tomato species into cultivated tomatoes in Table 2, along with information about their causal pathogens as footnotes. For example, the R genes/alleles conferring resistance to the begomoviruses that cause tomato yellow leaf curl diseases (called Ty genes), were found in wild tomato species. Most tomato advanced lines grown in tropical and subtropical regions of the world carry at least one source of Ty resistance genes (Ty-1/Ty-3) or multiple Ty genes (Ty-2, ty-5, Ty-6). The pyramiding of Ty genes into one line leads to broad and probably more durable resistance to begomoviruses. Likewise, R genes for late blight (caused by Phytophthora infestans, Ph genes) and fusarium wilt (caused by Fusarium oxysporum, I genes) also originated from wild tomatoes (Table 2). These genes are also frequently present in the modern tomato lines worldwide. Some important major QTLs (quantitative trait locus) such as Bwr and EB, conferring bacterial wilt (caused by Ralstonia spp.) and early blight (caused by Alternaria linariae) resistance, were first introgressed to adapted cultivated tomato cultivars from S. pimpinellifolium and later uncovered and genetically mapped. Some R genes (Rx-1, -2 and -3) for bacterial spot resistance (caused by several species of gram-negative bacteria in the genus Xanthomonas) were also derived from S. pimpinellifolium via cultivated tomato. Several R genes against important pathogens in tomato have mainly been introgressed, from the wild species S. pimpinellifolium, S. habrochaites, S. peruvianum, S. chilense and S. pennellii, into modern tomato varieties (Table 2). However, most tomato breeding programmes focus on S. pimpinellifolium due to its red fruit colour (Figure 1) and its close relationship to cultivated tomato, which allows breeders to easily obtain interspecific crosses. That is probably why most of the R genes have already been identified in this species.
Species |
R-genes’ contribution |
Disease/Insect pest |
References |
S. pimpinellifolium L. |
I and I-2 |
Fusarium wilt1 |
Stall and Walter (1965); reviewed in Chitwood-Brown, Vallad, Lee, and Hutton (2021) |
Ph-1, Ph-2, Ph-3 and Ph-5 |
Late blight2 |
Bonde and Murphy (1952); Gallegly and Marvel (1955); (AVRDC, 1994); Foolad, Merk, Ashrafi, and Kinkade (2006) |
|
|
EB-5 and EB-9 |
Early blight3* |
|
|
Bwr-6 and Bwr-12 |
Bacterial wilt4* |
|
|
Rx-1, Rx-2 and Rx-3 |
Bacterial spot5* |
Yu, Wang, Stall, and Vallejos (1995); reviewed in (Adhikari, Adhikari, Louws, & Panthee, 2020) |
|
Rx-4 |
Bacterial spot |
|
|
Sm |
Gray leaf spot6 |
|
|
Cf genes (except Cf-4 and Cf-5) |
Leaf mold7 |
Bailey (1950); reviewed in Scott and Gardner (2007) |
|
Sw-1(a and b), Sw-2, Sw-3 and Sw-4 |
TSWV8 |
(Finlay, 1953); Roselló, Díez, and Nuez (1998); (Zhu et al., 2017); reviewed in Qi et al. (2021) |
|
Pto |
Bacterial speck9 |
|
|
Cmm genes |
Bacterial canker10 |
Forster and Echandi (1972); Sotirova, Bogatsevska, and Stamova (1994); Sen, Manrique, Kabaş, and Visser (2021) |
S. habrochaites S. Knapp and D. M. Spooner |
Ph-4 |
Late blight |
|
Cf-4 |
Leaf mold |
||
|
Ty-2 |
TYLCV11 |
|
|
Tm-1 |
ToMV12 |
|
|
Ol-1/Ol-3, and Ol-5 |
Powdery mildew13 |
(Beek, G, & Lindhout, 1994); Huang, Putte, Meer, Meijer-Dekens, and Lindhout (2000); (Bai et al., 2005) |
|
Cmm genes |
Bacterial canker |
Forster et al. (1972); Francis et al. (2001); Coaker and Francis (2004); Sotirova et al. (1994) |
|
Rbcq genes |
Gray mould14 |
Have, Berloo, Lindhout, and Kan (2007); (Finkers et al., 2007; Finkers et al., 2007) |
S. peruvianum L. |
ty-5 |
TYLCV |
|
|
Sw-5 and Sw-6 |
TSWV |
Giordano, Avila, Charchar, Boiteux, and Ferraz (2000); Rosello, Ricarte, Diez, and Nuez (2001) |
|
Mi genes |
Root-knot nematodes15 |
Smith (1944); reviewed in El-Sappah et al. (2019) |
|
Tm-2, Tm-2 2 and Tm-2a |
ToMV |
(Soost, 1963); (Ganal & Tanksley, 1996) and Tanksley and Nelson (1996) |
|
Ve |
Verticillium wilt16 |
|
|
Frl |
Fusarium crown17 |
Vakalounakis, Laterrot, Moretti, Ligoxigakis, and Smardas (1997) |
S. chilense (Dunal) Reiche |
Ty-1/Ty-3a, Ty-4, and Ty-6 |
TYLCV |
Zamir et al. (1994); Ji, Schuster, and Scott (2007); Ji, Scott, Schuster, and Maxwell (2009); Gill et al. (2019) |
|
Sw-7 |
TSWV |
|
|
Cmm genes |
Bacterial canker |
|
|
Lv |
Powdery mildew |
Yordanov, Stamova, and Stoyanova (1975); Chunwongse, Doganlar, Crossman, Jiang, and Tanksley (1997) |
|
- |
Gray mould |
|
Species |
R-genes' contribution |
Disease/Insect pest |
References |
S. pennellii Correll |
I-3 and I-7 |
Fusarium wilt |
Catanzariti, Lim, and Jones (2015); (Gonzalez-Cendales, Catanzariti, Baker, Mcgrath, & Jones, 2016) |
|
Asc |
Alternaria stem canker18 |
|
|
Xv-4 |
Bacterial spot |
|
|
Acylsugar-related genes |
A wide range of insects |
(Leckie et al., 2016; Leckie, Jong, & Mutschler, 2012); Schilmiller, Charbonneau, and Last (2012) |
S. galapagense S. C. Darwin and Peralta |
Wf-1 and Wf-2 |
Whiteflies19 |
Firdaus et al. (2013); Santegoets, Bovio, Westende, Voorrips, and Vosman (2021) |
S. arcanum Peralta |
Ol-4 |
Powdery mildew |
|
Cmm genes |
Bacterial canker |
Crinò et al. (1995); Sotirova et al. (1994); Sen et al. (2013) |
|
S. neorickii D. M. Spooner, G. J. Anderson and R. K. Jansen |
V2 |
Verticillium wilt |
|
|
- |
Gray mould |
|
S. l. var. cerasiforme |
Cf-5 |
Leaf mold |
Dickinson, Jones, and Jones (1993); Dixon, Hatzixanthis, Jones, Harrison, and Jones (1998) |
|
ol-2 |
Powdery mildew |
|
|
|
|
|
1 Fusarium wilt caused by fungal pathogen Fusarium oxysporum (Schlecht. emend. Snyder & Hansen). 2 Late blight resistance caused by the oomycete Phytophthora infestans (Mont.) de Bary. 3 Early blight is caused by fungal pathogen Alternaria spp. 4 Bacterial wilt caused by the group of soilborne bacteria in the Ralstonia solanacearum species complex. 5 Bacterial spot caused by several species belonging to the genus Xanthomonas. It can be caused by Xanthomonas euvesicatoria ex Doidge, X. vesicatoria ex Doidge, X. perforans, and X. gardneri Šutic. *Early blight, bacterial wilt, and bacterial spot (Rx-1, Rx-2, and Rx-3) resistance genes are most likely derived from S. pimpinellifolium via cultivated tomato. 6 Gray leaf spot caused by fungal pathogen Stemphylium lycopersici (S. lycopersici). 7 Leaf mold caused by fungal pathogen Cladosporium fulvum (syn. Passalora fulva). 8 TSWV, tomato spotted wilt orthotospovirus (order Bunyavirales, family Tospoviridae, genus Orthotospovirus) is transmitted by Frankliniella occidentalis (Pergande) (Thysanoptera: Thripidae). 9 Bacterial speck disease caused by Pseudomonas syringae pv. tomato (Pst). 10 Bacterial canker caused by Clavibacter michiganensis subsp. Michiganesis. 11 TYLCV, tomato yellow leaf curl virus disease. TYLCV is caused by whitefly transmitted Geminiviruses (begomoviruses). Ty-1 and Ty-3 are allelic (Verlaan et al., 2013). 12 ToMV, tomato mosaic virus. ToMV is a member of the family tobamoviridae and belongs to the genus tobamovirus. 13 Powdery mildew can be caused by three species of biotrophic fungal pathogens; Oidium lycopersici, Oidium neolycopersici (syn. Pseoudoidium neolycopersici), and Leveillula taurica. Ol-1 and Ol-3 are allelic (Huang et al., 2000). 14 Gray mould caused by fungal pathogen Botrytis cinerea (teleomorph: Botryotinia fuckeliana). 15 Root-knot nematodes can be caused by Meloidogyne incognita, M. javanica, and M. arenaria. 16 Verticillium wilt caused by the biotrophic fungus Verticillium dahliae. 17 Fusarium crown rot caused by F. oxysporum f. sp. radicis-lycopersici. 18 Alternaria stem canker caused by fungal pathogen Alternaria alternate. 19 Whitefly (Bemisia tabaci) resistance. |
Challenges
The utilization of wild tomatoes in breeding programmes is not without a cost. CWRs generally show poor adaptation beyond their natural distribution range (Bohra et al., 2021). Furthermore, the introgressed gene from a wild relative into advanced lines may disrupt long-accumulated horticultural traits due to linkage drag (Tanksley et al., 1996). For example, S. galapagense has been identified as a source of insect-pest resistance (Rakha, Hanson, & Ramasamy, 2017; Vendemiatti et al., 2021). When it is crossed with cultivated tomatoes, the fruit size and setting reduce significantly, which are undesirable traits. Linkage drag can be removed by conducting backcrosses to the recurrent parents (cultivated tomato). Applications of DNA molecular markers (MAB, marker-assisted backcrossing) allow for the monitoring of the genome around the gene/locus of interest and the genetic background, speeding up the return to the recurrent parent genome (Tourrette, Falque, & Martin, 2021). Genome editing can also be used to remove the undesirable gene without having extensive backcrossing. For example, CRISPR-Cas9-based gene editing was used to overcome a linkage drag in tomato by editing the jointless-2 gene introgressed from S. cheesmaniae (Roldan et al., 2017). The World Vegetable Center is currently testing CRISPR-Cas9 to edit genes implicated with fruit size regulation in interspecific crosses between S. galapagense and cultivated tomato (Schafleitner, Lin, Khazaei, & Tsai, 2022).
Genomics-assisted breeding tools
Tomato wild relatives have more to offer.Bai, Kissoudis, Yan, Visser, and Linden (2018) stated that about 20 pathogens could be genetically controlled by resistance genes derived from a few wild species. The genome of some tomato wild species, including S. pennellii (Bolger, Scossa, Bolger, Lanz, & Maumuset, 2014; Schmidt, Vogel, Dentonet, Istace, & Wormitet, 2017), S. chilense (Stam et al., 2019), S. pimpinellifolium (Gramazio et al., 2020; Razali et al., 2018; Wang, Gao, Jia, Stravoravdis, & Hosmani, 2020) and S. lycopersicoides (Powell, Feder, Li, Schmidt, & Courtney, 2022) along with the pan-genome (Gao, Gonda, & Sun, 2019) have been assembled. These efforts have bolstered our knowledge and understanding of tomato wild species along with the genetics of resistance genes. Recent improvements in genomic resources have enabled us to track and genetically map the wild tomato genes in commercially adapted varieties (Anderson et al., 2021). The tomato community largely benefits from advanced, rich genomic resources (https://solgenomics.net/) and phenotyping tools; however, rapid generation technology (speed breeding) has not yet been developed. Robust DNA markers for major genes derived from wild relatives have been developed and widely applied in private and public breeding programmes worldwide (Foolad & Panthee, 2012; Hanson et al., 2016).
In the past, sources of important disease resistance genes in wild tomato relatives have been intensively investigated. However, the majority of R genes were discovered only in a few species (listed in Table 1). The other wild species that either are not easy to cross with cultivated tomatoes or are self-incompatible or allogamous have not contributed much to this journey (e.g. S. chmielewskii, S. corneliomulleri, S. huaylalloasense, S. juglandifolium, S. ochranthum, S. lycopersicoides and S. sitiens). Regarding these species, the literature only represents a few accessions of S. lycopersicoides being resistant to grey mould (caused by Botrytis cinerea) (Davis et al., 2009) or a few accessions of S. corneliomulleri being resistant to the TYLCV (Yan et al., 2018), but major genes/alleles from these species are yet to be reported. For some of these species, only a few accessions have been collected or are available in genebanks (Table 1). Among these species, some genomic studies were performed on S. sitiens (Chetelat et al., 2019) and S. lycopersicoides (Powell et al., 2022), which are potential sources of genes for adaptation to abiotic stresses (i.e. drought and heat stresses). Introgression lines were also developed from S. chmielewskii to study the accumulation of secondary metabolites in tomato fruit (Ballester et al., 2016).
Conclusions
Now that the scientific community has access to advanced tissue culture techniques, double haploidy protocols, modern phenotyping facilities, and genomic and bioinformatic tools, tomato wild relatives could be explored even more. This may provide new sources of genetic resources and R genes that could be used to pyramid new genes into one variety leading to broad and probably more durable resistance. Furthermore, ongoing advances in sequencing technology can be used to develop reference genome sequences for undiscovered tomato wild relatives, and the development of tomato pan-genomes will be a valuable strategy in harnessing the genetic diversity of these species. Additionally, genome editing enables de novo domestication strategies for the targeted use of tomato relatives (Zsögön et al., 2018). Thus, exploring the variation in tomato wild species could be an interesting topic for future studies.
Conflict of interest statement
The authors declare that they have no conflict of interest.
Author contributions
Original draft: Hamid Khazaei; review and editing: Hamid Khazaei and Adithya Madduri. All authors read and agreed to the published version of the manuscript.
Funding
Financial support was provided by the Foreign, Commonwealth and Development Office (UK) and by the long-term strategic donors to the World Vegetable Center, Taiwan: UK aid from the UK Government, the United States Agency for International Development (USAID), the Australian Centre for International Agricultural Research (ACIAR), Germany, Thailand, Philippines, Korea, and Japan. The authors thank Roland Schafleitner, Peter Hanson and Ya-ping Lin for their kind suggestions on the manuscript.