Leaf trichome diversity, acylsugar concentration, and their relationships to leaf area in Solanum galapagense
Abstract
Glandular trichomes are physical and chemical barriers used by some tomato wild relatives to confer resistance against insect pests and diseases transmitted by them. Solanum galapagense has been identified as one of the potential sources of insect pest resistance. The present study aimed to examine the trichome diversity and acylsugar concentration of 26 accessions of S. galapagense along with one cultivated tomato (S. lycopersicum) and one cherry tomato (S. l. cerasiforme) cultivar. The results revealed large phenotypic variation among S. galapagense accessions for all studied traits. The S. galapagense accessions had significantly higher trichome types IV density on the adaxial and abaxial surfaces of the leaf and greater acylsugar concentration but a smaller leaflet area than the cultivated tomato. The selected cherry tomato line represents greater trichome type IV density and acylsugar concentration than other groups. The acylsugar concentration was positively associated with trichome type IV but negatively associated with trichome type V on both leaf surfaces. DNA markers revealed the presence of two previously identified whitefly-resistance alleles in S. galapagense accessions. This study will support breeding programmes aiming to improve insect pest resistance in tomato cultivars using crop wild relatives.
Keywords
Acylsugar, crop wild relatives, leaflet area, S. Galapagense, tomato, trichomes
Introduction
Cultivated tomato (Solanum lycopersicum) is the most valuable vegetable crop by fruit weight globally, generating revenues of US$70 billion from 187 million tonnes of fresh fruit in 2020 (FAO, 2022). Improving fruit quality and yield through domestication in this crop, has led to the loss of important plant defence characteristics (Paudel et al., 2019), with tomato cultivation now heavily relying on pesticides to control biotic stresses (Dari, Addo, & Dzisi, 2016). The chemical treatments are not only costly, but they also harm the environment (Damalas & Eleftherohorinos, 2011). Developing resistant tomato cultivars could reduce the reliance on pesticides and their associated burden.
Tomato wild relatives are important sources of genetic diversity and are commonly used as reliable sources of resistance genes against biotic and abiotic stress (Ebert & Schafleitner, 2015; Khazaei & Madduri, 2022). Sources of resistance to insect pests have been identified in some tomato wild species, including S. galapagense, S. habrochaites, S. pennellii, S. cheesmaniae, and S. pimpinellifolium (Kennedy, 2003; Leckie et al., 2016; Rakha, Hanson, & Ramasamy, 2017; Schilmiller, Charbonneau, & Last, 2012; Vosman et al., 2018). Among them, S. galapagense has been identified as one of the most promising sources of insect pest resistance (Firdaus et al., 2012; Lucatti, Heusden, Vos, Visser, & Vosman, 2013). It has been the focus of most tomato breeding programmes aiming to improve biotic and abiotic stress resistance due to its close relationship to cultivated tomatoes (Vendemiatti et al., 2022). The S. galapagense species originates from the Galápagos Islands, an archipelago 1,000km west of Ecuador, where it formed a diverse range of phenotypes due to the islands’ unique ecosystem (Darwin, Knapp, & Peralta, 2003). While genetic studies revealed a narrow genetic diversity within the S. galapagense germplasm (Darwin, 2009; Pailles et al., 2017), it presents distinct morphological characteristics. These include yellow-green foliage, orange fruit at maturity, small seed size and highly divided leaves (Darwin et al., 2003; Fenstemaker, Sim, Cooperstone, Francis, & D, 2022).
Plants have developed a variety of defence mechanisms to counter biotic and abiotic stress conditions (Levin, 1973; Oksanen, 2018). One of these is the presence of fine outgrowths, called trichomes, on the surface of flowers, fruits, stems and leaves as physical and chemical lines of defence. Numerous studies have been conducted on the nature of these epidermal outgrowths, including function, quantification and effectiveness (Glas et al., 2012; Vendemiatti et al., 2022). Seven types of trichomes are characterized on plants, four are termed glandular due to the cells at their tip which can store and secrete metabolites (Luckwill, 1943). The presence of glandular trichome types IV and VI has been associated with higher insect pest resistance (Firdaus et al., 2013; Lucatti et al., 2013; Rakha et al., 2017; Zhang et al., 2020). These types of glandular trichomes deter insects through the release of secondary metabolites such as acylsugars, which cause behaviour changes and reduced survival in the arthropods that land on them (Antonious, Kochhar, Simmons, & M, 2005; Bleeker et al., 2011; Bleeker et al., 2012; Dias et al., 2016). In addition to acylsugars, other trichome metabolites such as terpenoids, methylketones and flavonoids play a key role in plant defence mechanisms (Huchelmann, Boutry, & Hachez, 2017). Two major genomic regions conferring whitefly resistance (Wf-1 and Wf-2), largely based on glandular trichomes type IV, have been identified in S. galapagense (accession id PRI95004; (Firdaus et al., 2013; Vosman et al., 2019)). Most likely, they regulate the formation of glandular trichome type IV on the leaf epidermis and subsequently control the accumulation of acylsugar on trichome type IV.
Trichome diversity and density, and their relationship with insect pest resistance have been investigated in tomato wild relatives, including S. galapagense (Firdaus et al., 2012; Lucatti et al., 2013; Rakha et al., 2017). An aspect deserving further attention is to harness the genetic diversity of morphological and biochemical characteristics of large germplasm of S. galapagense accessions and their relationships with leaf area. So, this study aims to uncover differences in trichome diversity, leaf characteristics and acylsugar concentration in this species. This is supported by the analysis of DNA markers associated with insect pest resistance phenotypes (Firdaus et al., 2013).
Materials and methods
Plant material
This study was conducted on 26 accessions of S. galapagense, one accession of cherry tomato (S. lycopersicum var. cerasiforme, abbreviated as S. l. cerasiforme), and one cultivated tomato (S. lycopersicum). Detailed information on accession number, origin and habitat at collection sites are presented in Table 1. All S. galapagense accessions originated from the Galápagos Islands, Ecuador (Figure 1). More than a third were collected on Isla Isabela, the largest island. This study is the first to screen accessions VI037867, VI037869, VI045262, VI057457, VI063173, VI063178, VI063179, VI063181, VI063182 and VI063183 for insect-pest resistance-related traits. The cultivated tomato is a breeding line from the World Vegetable Center (WorldVeg) carrying multiple tomato yellow leaf curl virus resistance genes (Ty-1/3 and Ty-2). The SM131 cherry tomato is a selection from accession VI063893 due to its high density of trichome type IV (unpublished data). All accessions were acquired from the WorldVeg genebank.
Seed treatment
Tomato seeds acquired from the WorldVeg genebank were treated with hydrogen chloride for 15 minutes and washed under running water. They were then treated with trisodium phosphate for one hour, washed under running water and dried in an incubator room at 60% for two days.
Growing conditions
Experiments were conducted in a glasshouse at the WorldVeg in Shanhua, Taiwan. Seeds were sown in a nursery on 25 February 2022, and after two weeks, were transplanted into 8-inch pots filled with cultivable soil collected from tomato fields. The pots were arranged in a randomized complete block design with four replicates. Plants were watered once a day in the morning and fertilized with a blend of 15–15–15 (N–P–K) at week four after transplanting. Relative humidity was about 80±15%. The temperature was set to 28±3°C during the day and to 22±2°C during the night.
Measurements
Leaflet area
The leaflet area was measured ten weeks after sowing using the third leaf from the apex. It was measured using a LI-3100 leaf area meter (LI-COR Inc., Lincoln, NE, USA). The same leaflet was also used for subsequent trichome and acylsugar measurements.
Trichome analysis
Analysis of leaf trichomes was conducted eight and ten weeks after sowing using a stereo microscope (Leica® M-Series Stereo microscopes, Ernst Leitz Wetzlar, GmbH, Germany). Leaf samples were collected at the third node from the apex using sterile forceps. The density of glandular trichome types I, IV, V and VI were measured from four randomly chosen leaflets within 1mm2.
Species |
Accession No. |
Origin |
Elevation (m) |
Other name(s) |
Habitat/phenotype |
---|---|---|---|---|---|
S. galapagense |
VI007099 |
Bartolome, Galápagos Islands |
15 |
LA0317, PI231257 |
Lava flow, amongst basalt rock, very arid, coastal arid zone |
|
VI037239 |
Isabela, Galápagos Islands |
40 |
LA0436 |
Sandy, near lava outcrop |
|
VI037241 |
Pinta, Galápagos Islands |
150 |
LA0526, SAL254 |
West side Abingdon island |
|
VI037339 |
Isabela, Galápagos Islands |
5 |
LA1401, PI365897 |
Among rocks in large beach with magma and lava flows at each end, collected few metres above tide line |
|
VI037340 |
Isabela, Galápagos Islands |
200 |
LA1408, PI379039 |
Ridge of Cape Berkeley volcano |
|
VI037867 |
Floreana, Galápagos Islands |
- |
LA1136, TL01054 |
Garnder near Floreana islet |
|
VI037868 |
Rabida, Galápagos Islands |
10 |
LA1137, TL01055 |
On cinder ash |
|
VI037869 |
Santiago, Galápagos Islands |
650 |
LA1141, TL01056 |
Interior walls of crater, purple fruit colour (Fenstemaker et al., 2022) |
|
VI045262 |
Santiago, Galápagos Islands |
- |
Selection from LA1141, TL01572 |
- |
|
VI057400 |
Fernandina, Galápagos Islands |
- |
LA483, 6201A, SAL241 |
- |
|
VI057408 |
Isabela, Galápagos Islands |
- |
Selection from LA1401 |
- |
|
VI057457 |
Galápagos Islands |
- |
LA3909 |
- |
|
VI063173 |
Bartolome, Galápagos Islands |
15 |
LA0317 |
Lava flow, amongst basalt rock, very arid |
|
VI063174 |
Isabela, Galápagos Islands |
30 |
LA0438, SAL192 |
Rocky basalt outcropping in first hills to W. (7km) from Villamil, 1km from coast |
|
VI063175 |
Isabela, Galápagos Islands |
20 |
LA0480A, SAL238 |
Along coast in bay facing Cowley Islet - not far from shore, in broken terrain without shade |
|
VI063176 |
Santa Cruz, Galápagos Islands |
- |
LA0528, SAL256 |
Academy Bay |
|
VI063177 |
Fernandina, Galápagos Islands |
580 |
LA0530, SAL258 |
Inside crater at edge |
|
VI063178 |
Galápagos Islands |
5 |
- |
- |
|
VI063179 |
Santiago, Galápagos Islands |
6 |
LA0747 |
In lava formation near shore |
|
VI063180 |
Santiago, Galápagos Islands |
3 |
LA0748 |
In lava formation |
|
VI063181 |
Isabela, Galápagos Islands |
4 |
LA0929 |
Growing in lava, roots in sand, above sea level |
|
VI063182 |
Isabela, Galápagos Islands |
4 |
LA0930 |
Growing in lava, roots in sand, same as LA 0929 |
|
VI063183 |
Bartolome, Galápagos Islands |
- |
LA1044 |
- |
|
VI063184 |
Isabela, Galápagos Islands |
400 |
LA1452 |
On the trail from Punta Ecuador to crater rim - mid-elevation in longer of two lava flows |
|
VI063185 |
Isabela, Galápagos Islands |
100 |
LA1627 |
Volcanic cone above Darwin's salt lake - likely Tagus Cove |
|
VI063187* |
Santiago, Galápagos Islands |
10 |
LA1411, PI379040 |
On soft bright red rock formation, margin of beach |
S. l. cerasiforme |
VI063893 |
Fernandina, Galápagos Islands |
- |
SM131 |
- |
S. lycopersicum |
CLN3682C |
Breeding line |
- |
AVTO1424 |
Pedigree: CLN3682F1-10-3-4-27-3-16 |
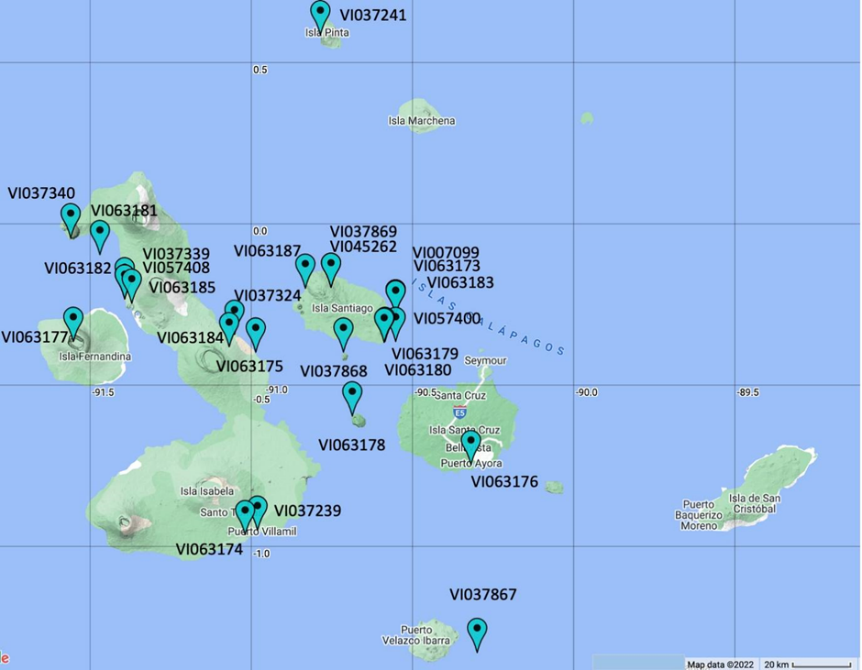
The number of trichomes was counted from four different microscopic fields at 5X magnification and converted to the number per mm2 using a standard scale. The identification of trichome types on the leaf surface followed a schematic drawn by Luckwill (1943). After measuring trichomes on the adaxial surface, the leaflets were flipped to measure the trichomes on the abaxial surface.
Acylsugar concentration
Analysis of acylsugar content was conducted at eight and ten weeks after sowing. Polyethylene vials were used to collect four 3±1cm lateral leaflets from each plant at the third node from the apex. Samples were dried in an incubator at 29°C for three days before washing them with 3ml methanol. Of this suspension, 100μl was added to 100μl 6M Ammonium Hydroxide in 96 well ELISA plates with two biological replicates, following a protocol developed by Martha Mutschler (Savory, 2004). The samples were incubated overnight and left to dry under the hood for three days before adding 200μl PGO reagent to each well and placing it on an orbital shaker. After three hours, absorbance values at 490nm were measured using BioTek’s uQuant (Agilent Technologies Inc., Santa Clara, CA, USA) and converted into acylsugar concentration using a sucrose standard curve.
DNA extraction and DNA marker assay
Genomic DNA was extracted from 10-week-old plants using the CTAB method (Doyle & Doyle, 1990). The Wf-1 and Wf-2 detailed marker sequences presented in Firdaus et al. (2013) were used for genotyping the studied germplasm for the presence/absence of corresponding bands. The term ‘Wf’ stands for whitefly and represents markers previously identified for whitefly resistance. These markers are located in tomato chromosomes 2 and 9, respectively. Purified DNA samples were digested with restriction enzymes DDeI and HpyCH4IV for Wf-1 and Wf-2 markers, respectively. Digested samples were amplified along with marker-specific primers using PCR reactions as described by Mahfouze and Mahfouze (2019). The PCR-amplified samples were run on a 5% acrylamide gel for 30 minutes at 100V and stained using an ETBR-out stain. The gel was scanned in a Bio-1000F scanner, and the amplified bands visualized using Microtek MiBio Fluo software (both from MicroTek International, Inc., Hsinchu, Taiwan).
Statistical analysis
The R statistical package (R Core Team, 2021) was used for data analysis. Correlation analysis was performed to determine the relationships between morphological measurements. The dataset was subjected to a one-way analysis of variance (ANOVA), and the SEM (standard error of means) was calculated. Principal component analysis (PCA) was employed to illustrate relationships between accessions and leaf morphological measurements. The online mapping tool at maps.co was used to plot the coordinates of accessions in Figure 1 (https://maps.co/). The geographic coordinates of S. galapagense accessions were obtained from the Tomato Genetics Resource Center (C.M. Rick TGRC, https://tgrc.ucdavis.edu/) and the WorldVeg (https://genebank.worldveg.org/#/) genebank databases.
Results
Trichome densities varied significantly among studied germplasm (Table 2 and Supplemental Table 1). Trichome type IV density ranged from 6.3 to 13.5 for the abaxial and 0.7 to 10.9 for the adaxial surface of S. galapagense accessions, while the cultivated tomato (CLN3682C) had none on either surface. Accessions VI057408, VI063174, VI063177, VI063185 and VI057400 had the greatest number of trichome type IV on both surfaces. The cherry tomato (VI063893) had 22% greater (on both surfaces) trichome type IV compared to the average values of S. galapagense accessions. Within S. galapagense accessions, trichome type VI varied from 0.4 to 2.7 on the abaxial and 0.3 to 2.8 on the adaxial side. For the abaxial surface, this was 30% and 14% lower than cultivated and cherry tomato cultivars, respectively. For the adaxial surface, it was 94% lower and 30% higher than cultivated and cherry tomatoes, respectively (Table 2). Most studied accessions had fewer trichomes on the adaxial than on the abaxial side, with 13% less for type IV and 40% less for type VI. Comparing 8- and 10-week trichome phenotyping at the abaxial surface, S. galapagense trichome densities remained stable with a 3% increase for type IV, and a 12% decrease in type VI.
|
|
Trichome types per mm 2 – Abaxial |
Trichome types per mm 2 – Adaxial |
Acylsugar (µmol/g) |
Leaflet area (cm 2 ) |
||
Species |
Accession No. |
IV |
VI |
IV |
VI |
|
|
S. galapagense |
VI063181 |
8.0±5.7 |
1.6±1.3 |
7.1±1.6 |
2.6±0.9 |
58.02±8.32 |
8.01±1.84 |
|
VI037869 |
10.5±1.6 |
0.6±0.4 |
9.1±1.8 |
0.2±0.5 |
57.68±15.42 |
6.12±2.67 |
|
VI045262 |
11.3±1.2 |
1.0±0.4 |
8.7±1.3 |
0.4±0.1 |
54.02±11.27 |
5.95±1.56 |
|
VI057408 |
13.5±1.3 |
1.0±0.5 |
9.1±0.7 |
2.8±0.7 |
46.64±12.57 |
3.83±0.65 |
|
VI063184 |
10.1±1.1 |
1.1±0.7 |
7.5±1.8 |
1.4±0.3 |
45.56±4.43 |
5.92±0.95 |
|
VI063187 |
9.2±1.3 |
1.0±0.8 |
8.6±1.4 |
0.5±0.2 |
44.25±9.63 |
3.81±1.45 |
|
VI063185 |
12.0±0.5 |
1.5±0.3 |
9.0±3.0 |
1.1±0.9 |
43.49±5.71 |
5.15±0.45 |
|
VI057400 |
11.8±0.9 |
0.9±0.6 |
10.8±0.8 |
0.3±0.3 |
43.07±4.51 |
4.40±0.18 |
|
VI063177 |
12.1±1.0 |
1.1±0.5 |
9.7±2.5 |
0.4±0.1 |
41.75±11.28 |
5.15±1.46 |
|
VI037241 |
10.1±1.4 |
0.3±0.1 |
6.0±1.4 |
0.5±0.2 |
34.00±0.01 |
2.88±0.17 |
|
VI037339 |
7.7±0.6 |
0.7±1.5 |
9.3±2.5 |
0.3±0.0 |
32.40±0.01 |
6.89±0.0 |
|
VI063182 |
11.1±1.9 |
0.8±0.3 |
8.5±1.0 |
0.6±0.3 |
32.39±6.25 |
6.15±1.78 |
|
VI057457 |
10.0±2.7 |
2.5±1.5 |
9.0±1.2 |
0.3±0.0 |
31.87±6.97 |
2.65±1.01 |
|
VI063174 |
12.4±0.9 |
1.0±0.5 |
10.8±1.5 |
1.2±0.6 |
30.58±8.12 |
6.81±3.33 |
|
VI063179 |
6.2±5.5 |
2.0±1.8 |
4.3±0 |
1.3±0.0 |
25.20±1.86 |
6.59±0.83 |
|
VI037340 |
11.3±1.3 |
1.1±0.9 |
8.5±1.5 |
0.8±0.6 |
24.65±2.52 |
7.07±1.03 |
|
VI037239 |
9.7±0.7 |
1.2±0.7 |
9.5±1.1 |
1.1±0.2 |
24.60±0.01 |
6.60±1.2 |
|
VI063175 |
12.0±1.4 |
2.6±1.6 |
7.5±1.1 |
1.6±1.4 |
22.53±5.67 |
4.60±1.62 |
|
VI037868 |
10.6±2.3 |
1.8±0.4 |
9.5±1.6 |
1.4±0.5 |
22.08±5.05 |
5.40±1.43 |
|
VI063183 |
10.3±1.5 |
2.4±1.4 |
7.2±1.7 |
0.8±0.1 |
22.07±1.52 |
2.43±1.66 |
|
VI037867 |
9.0±0.4 |
1.5±0 |
7.7±1.8 |
0.7±0.5 |
18.46±1.81 |
5.17±0.38 |
|
VI063176 |
9.3±5.3 |
0.9±0.9 |
7.7±5.4 |
0.5±0.5 |
16.06±4.36 |
4.37±1.86 |
|
VI063173 |
11.6±0.5 |
2.4±1.6 |
9.4±2.8 |
1.6±1.2 |
15.29±4.70 |
5.46±1.92 |
|
VI063180 |
10.3±1.8 |
1.4±0.5 |
8.1±2.5 |
0.3±0.3 |
15.23±2.15 |
7.07±1.77 |
|
VI063178 |
10.0±1.3 |
1.6±1.5 |
8.7±2.7 |
1.1±0.3 |
14.8±3.61 |
5.32±0.98 |
|
VI007099 |
6.6±2.9 |
1.6±0.5 |
0.6±0.8 |
1.8±0.5 |
5.43±5.88 |
9.15±5.5 |
|
Range (S. galapagense) |
6.2–13.5 |
0.3–2.6 |
0.6–10.9 |
0.2–2.8 |
5.43–58.02 |
2.43–9.15 |
|
Mean (S. galapagense) |
10.3±1.7 |
1.4±0.6 |
8.2±2.0 |
1.0±0.7 |
31.6±14.9 |
5.50±1.59 |
S. l. cerasiforme |
VI063893 |
12.6±1.5 |
1.6±0.4 |
10.0±2.2 |
0.7±0.3 |
21.02±15.93 |
6.10±0.94 |
S. lycopersicum |
CLN3682C |
0.0 |
2.0±0.8 |
0.0 |
4.6±2.3 |
0.94±0.23 |
14.36±3.64 |
SEM |
|
1.7 |
0.2 |
1.6 |
0.2 |
16.95 |
1.3 |
Acylsugar concentration varied significantly (P < 0.001) among S. galapagense accessions, ranging from 5.43 to 58.03 µmol/g. The cultivated tomato cultivar (CLN3682C) showed a very low acylsugar concentration of 0.94µmol/g and the cherry tomato (VI063893) showed a moderate level of 21.02µmol/g (Table 2). On average, 10-week-old S. galapagense accessions had 45% greater acylsugar concentrations than 8-week-old plants. Accessions VI063181, VI037869 and VI045262 had the highest concentration of acylsugar, all above 50µmol/g.
The leaflet area varied significantly (P < 0.001) among S. galapagense accessions, ranging from 2.43 to 9.15cm2. Leaflet area was significantly greater for cultivated tomato, with 14.36cm2 than for all S. galapagense accessions. On average, cherry tomato leaflets were 10% larger than S. galapagense accessions (Table 2).
Correlations between trichome types and acylsugar concentration are presented in Table 3. Acylsugar concentration was positively associated with trichome type IV and negatively associated with trichome type V. The negative correlation between acylsugar and trichome type VI was only significant at the 10-week-old plant stage. In addition, leaflet area was negatively correlated with trichome IV density of abaxial surface (P < 0.001, Figure 2A) and acylsugar concentration (P = 0.078, Figure 2B).
Trichome type |
AS (8-week-old) |
AS (10-week-old) |
Type I |
-0.140 |
-0.238 |
Type IV |
0.473* |
0.410* |
Type V |
-0.540** |
-0.459* |
Type VI |
-0.347 |
-0.471* |
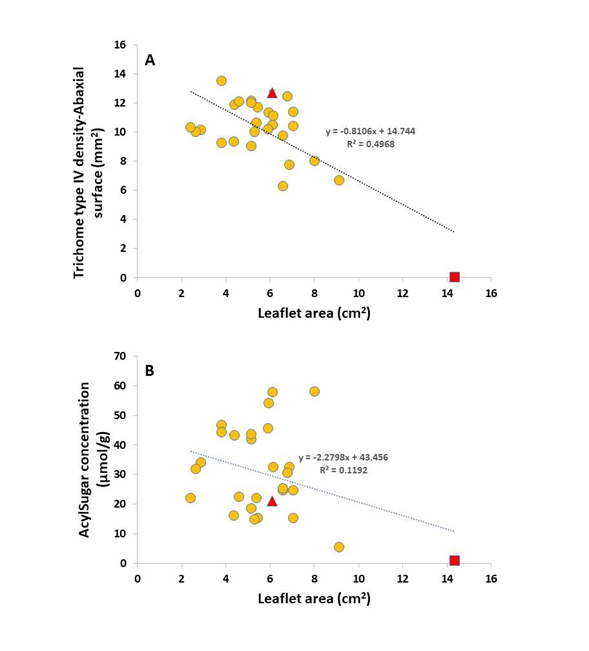
The PCA plot (Figure 3) showed three clusters based on acylsugar, trichome type and leaf area measurements. The first group consisted of S. galapagense accessions and the cherry tomato genotype (VI063893), which was correlated with high acylsugar concentration as well as high levels of trichome type IV on the adaxial surface. The cultivated tomato (CLN3682C) was separated from S. galapagense group mainly due to its greater leaflet area and high levels of trichome type V. One of the S. galapagense accessions, VI007099, was grouped closer to the cultivated tomato mainly due to its larger leaflet area (its leaf morphology was similar to cultivated tomatoes) compared to other accessions from the Galápagos Islands.
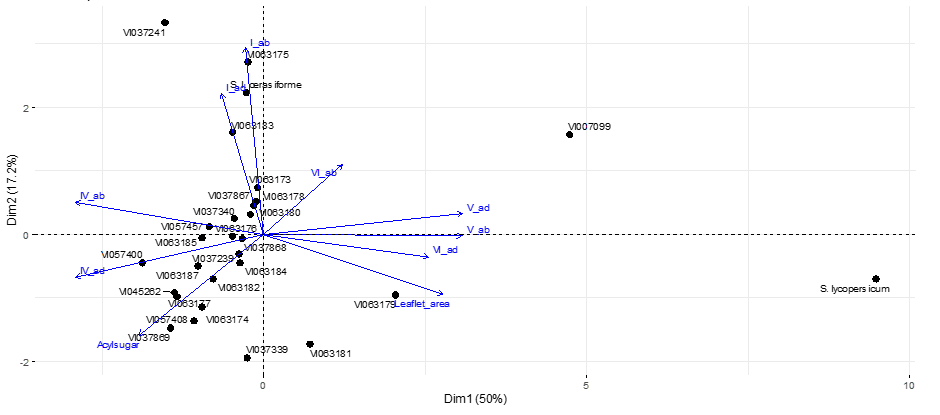
The DNA marker results are presented in Supplemental Table 3. All accessions of S. galapagense showed both resistance bands at 46 and 40bp for the Wf-2 marker (indicated by ‘++’) while both S. lycopersicum cultivars (CLN3682C and VI063893) showed the susceptible band at 87bp (indicated by ‘-‘). The Wf-1 marker showed a resistance band at 139bp (indicated by ‘+’). Both S. lycopersicum cultivars showed the susceptible band at 157bp (indicated by ‘-‘).
Discussion
In this study, we screened a large germplasm collection of S. galapagense accessions revealing a considerable phenotypic variation in glandular trichome density and acylsugar concentration. Our results indicated that more than 50% of S. galapagense accessions had acylsugar concentration more than 30µmol/g – on average, 34 times greater than the studied S. lycopersicum (CLN3682C) genotype. However, not all S. galapagense had high concentrations and trichomes types IV and VI density. There was a 12-fold difference between the greatest and lowest S. galapagense accessions for acylsugar concentration. However, only a 2-fold variation was observed for trichome type IV density in this species. Our results also showed that tomato plants with smaller leaves had higher densities of trichome type IV.
The selection of tomato plants with higher densities of glandular trichomes IV and VI has shown to be an effective criterion for obtaining superior resistance to insect pests. This has been widely proven in resistance to spider mites (Tetranychus urticae, Andrade et al. (2017); Rakha et al. (2017); Souza-Marinke et al. (2022)), whitefly (Bemisia tabaci, Lucatti et al. (2013); Rakha et al. (2017)), thrips (Escobar-Bravo, Klinkhamer, & Leiss, 2017), and cabbage looper caterpillar (Mymko & Avila-Sakar, 2019). These studies generally attribute insect-pest resistance to the excretion of acylsugars by the tip of glandular trichome type IV, thereby acting as a biopesticide.
Several studies have reported genetic diversity for trichome density and acylsugar concentration in tomato (Baier, Resende, Faria, Schwarz, & Meert, 2015; Lucatti et al., 2013; Rakha et al., 2017). These have allowed tomato breeding programmes globally to exploit the available diversity and improve insect-pest resistance cultivars. We screened a relatively larger number of S. galapagense accessions (26) compared to previous studies, which only characterized 10 (Rakha et al., 2017) or 11 accessions (Lucatti et al., 2013). Our study revealed a wider variation for trichome type IV, however, smaller values for acylsugar concentration compared to a similar study by (Rakha et al., 2017). The cherry tomato genotype (VI063893) was previously characterized by high trichome type IV density (unpublished data). Our results confirm that its trichome type IV density was higher than 96% of studied S. galapagense accessions (Table 2). This cherry tomato genotype may be used as a source of insect resistance in cherry and cultivated tomato germplasm.
A moderate positive correlation (P < 0.05) was observed between acylsugar concentration and trichome type IV at two different sampling times. Previous studies also reported a similar trend (Lucatti et al., 2013; Rakha et al., 2017). A possible explanation for this could be the poor phenotyping of trichomes under the microscope, as counting the number of trichomes is an inherently delicate task. This difficulty highlights the need for high throughput methods to measure trichomes. Another explanation for the lack of strong correlation between trichome IV density and acylsugar could be that acylsugar production is not solely linked to trichome density but also their metabolic activity, whereby the same trichome types in different accessions produce varying levels of acylsugar (Bergau, Bennewitz, Syrowatka, Hause, & Tissier, 2015; Zhang, Thacker, & Snyder, 2008). Following this reasoning, isolated trichomes could be tested for metabolic activity through GC-MS as described for L. hirsutum by Fridman et al. (2005).
A negative correlation was observed between trichome type IV and leaflet area, a trend that has been reported in other plant species, including S. berthaultii Hawkes (Pelletier, 1990) and silver birch (Betula pendula Roth) (Lihavainen et al., 2017).Mymko and Avila-Sakar (2019) reported that unexpanded leaves had greater trichome density and resistance than expanded (larger) leaves at different growth stages of tomatoes. In our study, leaflet area was one of the main drivers in allocating tomato species into three different groups (Figure 3). Accession VI007099 had the greatest leaflet area and lowest trichome density and acylsugar concentration among S. galapagense and presented leaf morphology between wild and cultivated tomatoes. On the other hand, accession VI063181 had the second-largest leaflet area among S. galapagense accessions but also the greatest acylsugar concentration among all accessions. This controversy was also evident by the weak correlation between leaflet area and acylsugar concentration (Figure 2). These results suggest that acylsugar concentration may not be derived by leaf size and trichome type IV in S. galapagense germplasm.
No clear pattern between leaf trichome measurements and accessions’ geographical origin in the Galápagos Islands was observed. The only accession from Pinta Island, VI037241, was separated from other S. galapagense. Pinta Island is the northernmost of the main islands and has a semi-arid ecosystem (Hunter & Gibbs, 2014). The main driver for the deviation of other S. galapagense from the core cluster (Figure 3) was leaflet area (VI063181, VI063179, VI037339 and VI007099) and higher trichome type VI density (VI063175 and VI063183). The three accessions with the highest trichome type IV density were all found among volcanic rock with VI057408 and VI06374 found within 1km of the sea on Isla Isabela and VI063177 inside a volcanic crater on Isla Fernandina. An explanation for this correlation could be the presence of silicon in volcanic soil as it has been shown to boost plants' resistance to pests by accumulating magnesium at the base of trichomes (Ma, 2004).
Some S. galapagense accessions originating from the Galápagos Islands have been exposed to dry and saline growing conditions (Pailles et al., 2020) and high insect pressure (Peck, 2008), thus may represent a generous source of alleles that can be explored to improve biotic and abiotic stress. As this species can easily hybridize with cultivated tomatoes (Rick, 1961), they have been used as donors for stress tolerance genes, which could be transferred into commercial varieties by introgression breeding (Zamir, 2001). For example, VI037339 (LA1401) and VI007099 accessions have already been utilized as donors of high trichome IV density into modern cultivated tomato cultivars through interspecific crosses (Andrade et al., 2017; DaSilva et al., 2019; Rakha et al., 2017; Vendemiatti et al., 2022). However, in this study, these accessions were not among those with the highest trichome type IV density and acylsugar concentration. This could be due to the genotype-by-environment interactions as the experiments were carried out under different growing conditions.
From the analysis of DNA markers, we could see that most S. galapagense accessions were homozygous for Wf-1 and Wf-2 but neither S. lycopersicum cultivars. This suggests that the morpho-chemical measurements in this study were linked to the genetic background of S. galapagense accessions. However, the cherry tomato genotype VI063893 (SM131), which showed high levels of trichome type IV and acylsugar, did not amplify either band of the DNA markers. This was not surprising as those DNA markers were developed from an interspecific population derived from S. galapagense (Firdaus et al., 2013). A potential reason why the cultivated tomato accession (CLN3682C) did not show the resistance markers is that the related genes may have been lost during domestication. We conclude that Wf-1 and Wf-2 may be more suitable to be used in genetic materials derived only from S. galapagense. The other possibility is that the source(s) of resistance in VI063893 is non-allelic to Wf-1 and Wf-2.
In conclusion, our study focused on screening a large S. galapagense germplasm, supporting breeding programmes aiming to improve insect-pest resistance in tomatoes using crop wild relatives. The ultimate goal is to develop tomato cultivars with insect-pest resistance-related traits that help farmers reduce pesticide use and produce a high-quality and chemical-free tomato crop. The glandular trichome density and chemistry are highly affected by the genotype by environment interactions (Wang, Shen, Meng, Tan, & Lv, 2021). This needs to be considered when selecting these traits under field conditions.
Supplemental data
Supplemental Table 1. Mean ± SD (standard deviation) for trichomes I and V measurements (abaxial and adaxial surfaces)
Supplemental Table 2. Trichome density and acylsugar concentration at 8-week-old seedlings of S. galapagense Supplemental Table 3. DNA marker assay for B. tabaci whitefly resistance alleles Wf-1 and Wf-2. N, missing dataData availability
The data that support this study will be shared upon reasonable request to the corresponding author.
Conflicts of interest
The authors declare no conflicts of interest
Author contributions
IH and HK did the conceptualization, data curation and formal analysis, led the methodology, and created the original draft of the manuscript.
Acknowledgement
We would like to thank Yun-che Hsu (Grace) and Jean Lin for their kind assistance during the experiments. We also thank Dr Roland Schafleitner (Flagship Leader, Vegetable Diversity & Improvement) and Dr Maarten van Zonneveld (Genebank manager) for their valuable suggestions during the experiments. In addition, the first author would like to thank the National Cheng Kung University (NCKU), Taiwan for its support.
Funding
Financial support was provided by long-term strategic donors to the World Vegetable Center: Taiwan, UK aid from the UK government, the United States Agency for International Development (USAID), the Australian Centre for International Agricultural Research (ACIAR), Germany, Thailand, Philippines, Korea, and Japan.