Optimum contribution selection (OCS) analyses prompted successful conservation actions for Faroese horse population
Abstract
The Faroese horse, an endangered indigenous horse breed, is a part of the cultural and societal heritage of the Faroe Islands. Population history describes a severe bottleneck, prompting for quantification of the genetic diversity (level of inbreeding, probability of gene origin, effective population size) and assessment of sustainable conservation potential (Optimum Contribution Selection, OCS) of the Faroese horse population. The pedigree completeness (PCI) of the Faroese horse is adequate for a realistic estimation of the level of inbreeding (PCI5 = 0.96). In concordance with the known population history, the average inbreeding is exceptionally high; in the last cohort, it was equal to 26.8%. An estimate of the effective population size, based on individual increase in inbreeding and coancestry, accounting for the whole population history, was eight. OCS offers a tool to understand and control the increase in the average relationships in the population. Within a fixed number of matings, the repetitive use of stallions resulted in the lowest level of average relationships. Successful follow-up of mating schemes planned together with a holistic assessment of the suitability of an individual as a breeding candidate, will minimize the increase in inbreeding in future generations and maximize the possibility to increase the census size of the Faroese horse population.
Keywords
Conservation, native breed, horse, effective population size, optimum contribution selection
Introduction
The Faroese horse (Føroyska rossið in Faroese) represents an important part of the agricultural history and cultural integrity of the Faroe Islands. According to the Domestic Animal Diversity Information System (DAD-IS) of the Food and Agriculture Organization of the UN (FAO), the breed status is defined as critical and at risk. The history of the Faroese horse is not well documented and there is ambiguous evidence of the origin of the Faroese horse. Most likely the Faroese horse descended from Scandinavian and/or Celtic horse breeds brought to the Faroe Islands by settlers in 500–800 CE (Bjørk, 1984; Joensen, 2019). In written sources, the Faroese horse and its use are first described in the 1600s (Bjørk, 1984; Joensen, 2019). Despite their small size, the Faroese horses were traditionally used for carrying heavy loads, only seldom used for riding, and never as draught horses as there were no suitable roads for that (Bjørk, 1984). Horses were only brought to the villages when they were needed as a working force to carry manure to the fields, peat to the houses or during the harvest of hay and grain (Bjørk, 1984). Outside of these periods of gruelling work, horses were kept free ranging without supplemental feed all year round. Consequently, the Faroese horse developed into a small (115–125cm at withers), strong, resilient horse well adapted to the demanding climate, terrain and vegetation of the Faroe Islands. At its highest, the population size of Faroese horses has been approximately 800 individuals (Bjørk, 1984).
In the late 19th century, a shift of labour from agriculture to fisheries and the mechanization of agriculture decreased the importance of horses as working power for agriculture. At the same time, the Faroese horse became a valuable export commodity; a large number of Faroese horses were exported to the British Isles as pit ponies for the coal mining industry (Bjørk, 1984; Joensen, 2019). The need for pit ponies was increased when the use of women and children as workforce in the mines was made illegal. The reduced population size and decreased interest in pure Faroese horses set off demand for the importation of foreign breeds to the Faroe Islands. Consequently, Norwegian Fjord horses and Icelandic horses were imported and interbred with Faroese horses. Crossbreeding increased the height at withers (125–132cm) and improved the working ability of the horse, but had a deteriorating effect on the overwintering ability (Bjørk, 1984). Although the documentation of the population management is deficient, heavy restrictions on stallion use during the 1930s when foreign breeds were strongly favoured, are described in literature (Bjørk, 1984). By the 1960s, the Faroese horse population was close to extinction with only five individuals alive.
Currently, a small population of Faroese horses is maintained on the Faroe Islands by hobby breeders. Today, this unique breed is used for recreational purposes and tourism. The current population stems from a few horses born between the 1940s and 1960s. In 1978, the organization Felagið Føroysk Ross was established to bring together people dedicated to conserving this native breed. The genetic variation in the Faroese horse population using 12 microsatellite markers was earlier assessed by Mikko, Leivsson, and T (2004). As expected, the population had low genetic variation but did not show any apparent signs of inbreeding. In recent years, the interest in the sustainable conservation of Faroese horses has greatly increased. Given the traditional knowledge of the population history, and due to the limited success in increasing the population size, there is a need for a knowledge-based management plan for this unique horse breed.
Genetic diversity describes the range of different traits inherited in a population. Genetic diversity is a prerequisite for selective breeding and critical for a population’s adaptation potential in changing environments. Endangered local breeds typically have small census sizes and low genetic diversity. Consequently, minimization of the rate of inbreeding is imperative for the sustainable conservation of these breeds. Optimum Contribution Selection (OCS) is a method that enables maximization of the selected parents' genetic level and simultaneously constrains their average coancestry at a desired level (Henryon, Ostersen, Ask, Sørensen, & Berg, 2015; Meuwissen, 1997). OCS is used in many conservation programmes, and for example, suggested by Nielsen and Kargo (2020) as part of the conservation strategy to save the endangered Jutland horse.
Our hypothesis was that the Faroese horse expresses extremely low genetic diversity and high levels of inbreeding, and that further rapid increase in inbreeding can be circumvented by using OCS. To test this hypothesis, we conducted a pedigree analysis of the Faroese horse population to assess the level of genetic diversity. Effective population size (Ne), the key parameter for describing genetic diversity in animal populations, was estimated with several methods. Additionally, we used OCS to investigate the possibilities for sustainable management of this horse breed. Based on these results, management recommendations were drafted.
Materials and methods
Data
Pedigree data, comprising horses born between 1917 and 2016, were obtained from the Felagið Føroysk Ross. Out of the 178 individuals, 87 were males and 91 females.
In the 1960s the population of Faroese horses experienced a severe bottleneck with only five individuals alive (Figure 1). These were one stallion born in 1962 and four mares born in 1946, 1952, 1956 and 1958. One of the mares is the dam of two of the other dams, both being a result of a sire–daughter mating. One of the mares alive in the 1960s is unrelated to the other four individuals. The number of horses born per year is presented in Figure 2. A very low number of foals were born yearly until the recent years when invigorated national efforts for the sustainable conservation of the Faroese horse have resulted in increased population size (Figure 2). Between 2000 and 2016, a total of 92 foals were born, out of which 66 were alive at the time of the study (December 2016). It is worth mentioning that additional 25 foals have been born between 2017 and August 2019.
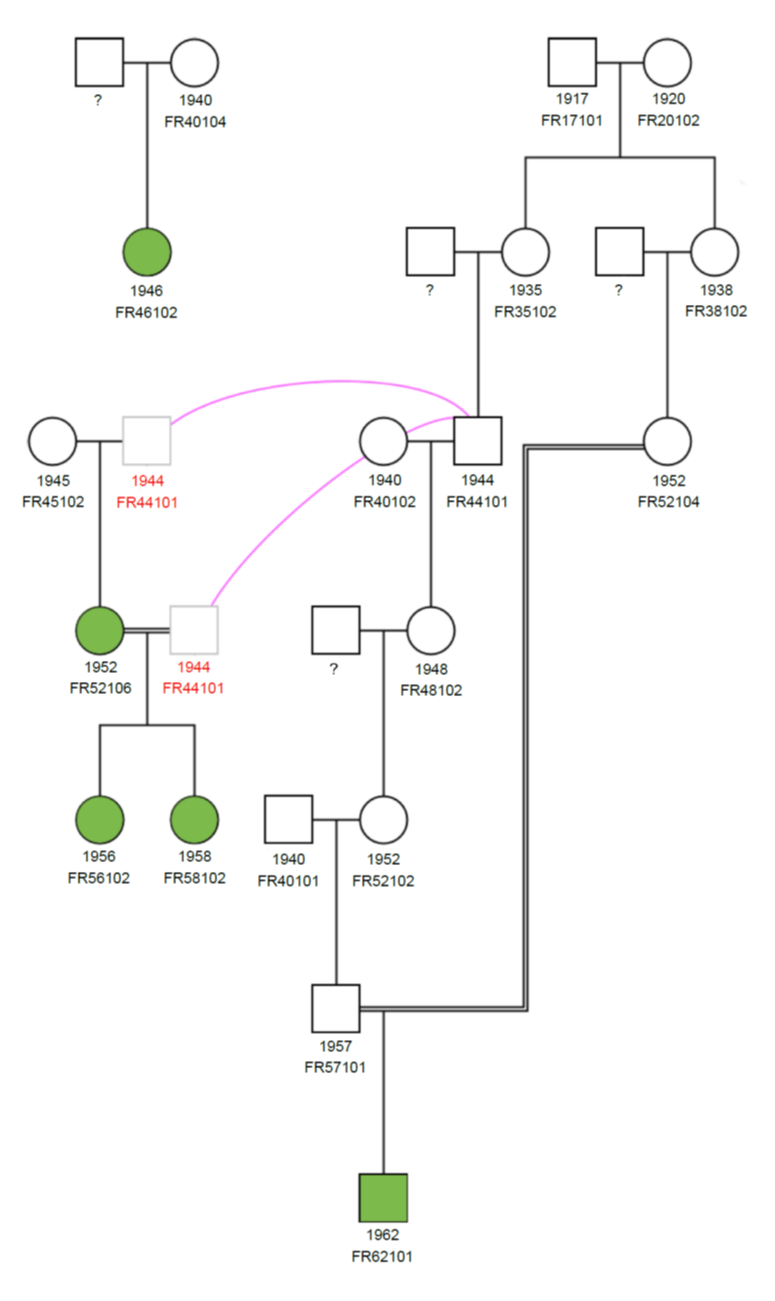
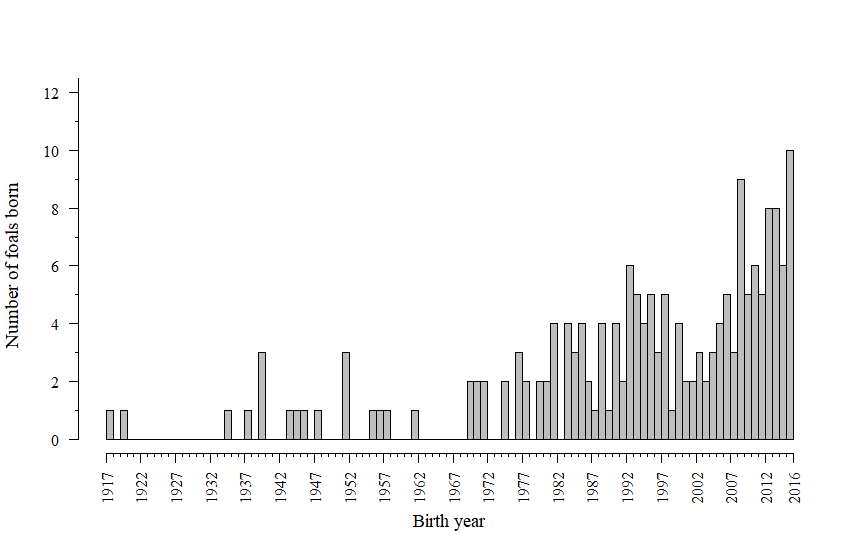
Statistical methods
Population analyses
Pedigree completeness, level of inbreeding and additive genetic relationships were calculated using EVA 2.1 (Berg, Nielsen, & Sørensen, 2006; Henryon et al., 2015). Two birth years were combined into biannual cohorts from the year 1991 and onwards due to the low number of foals born per year. Estimates of effective population size and parameters derived from the probabilities of gene origin were obtained by ENDOG v.4.8 (Gutiérrez & Goyache, 2005). More specifically, different methods were used to derive and thereafter effective population was computed as , using the same estimators of rate of inbreeding as in a previous study on Norwegian Lundehund (Kettunen, Daverdin, Helfjord, & Berg, 2017).
In short, three regression coefficients of the individual inbreeding coefficients on the number (method identification in parentheses) of i) complete generations (Ia), ii) maximum number of generations (Ib) and ii) equivalent generations (Ic) traced back to founders for each individual, were computed to give information on the lower, upper and 'real' limits of . Further, two regression methods were used to approximate ; first, the regression coefficient of the individual inbreeding coefficients on birth years (II), and second the regression coefficient of the inbreeding coefficients regressed on the number of equivalent generations (IV). The average generation interval (Gutiérrez et al., 2003) was used to define the increase in inbreeding between two generations. In two other methods values of were regressed either on birth years (IIIa) or complete generation equivalents (IIIb) (Pérez-Enciso, 1995). Additionally, individual increases in inbreeding (V) (Gutiérrez, Cervantes, & Goyache, 2009; Gutiérrez, Cervantes, Molina, Valera, & Goyache, 2008) and increase in coancestry (VI) for all pairs of individuals in the reference population (Cervantes, Goyache, Molina, Valera, & Gutiérrez, 2011) were used for estimation of Ne and the standard error of the estimated Ne. Additional details of the methods used are presented in Kettunen et al. (2017) and references therein.
Parameters derived from the probabilities of gene origin, that is the effective number of founders (fe), ancestors (fa), non-founders (fne) and the founder genome equivalent (fge) were used to describe the genetic variability in the Faroese horse population. The definition and calculation of these parameters can be found in literature (Boichard, Maignel, & Verrier, 1997; Caballero & Toro, 2000; Lacy, 1989; Lacy, 1995). These parameters were used to calculate the degree of genetic diversity relative to the base accounting for loss of diversity due to unequal founder contribution, bottlenecks and genetic drift (Kettunen et al., 2017). Parameters fe , fa and fne were obtained from ENDOG whereas fge was calculated as , where was the average coancestry of all living individuals (Caballero et al., 2000).
Optimum contribution selection
EVA 2.1 software was used for the OCS analyses (Berg et al., 2006; Henryon et al., 2015). Horses born between 1995 and 2014 were considered breeding candidates. Individuals with known health or reproductive problems were excluded as breeding candidates prior to OCS analyses. This resulted in a total of 24 males and 28 females being available for breeding. Genetic contributions were optimized for 6 to 12 matings, constraining the number of matings allowed for each stallion from 1 to 4. Genetic contributions were optimized using default algorithm parameters (Supplemental data) with full penalty on average relationships; this equals zero weight on genetic merit with all focus on minimization of the average relationships.
Results and discussion
Pedigree completeness and inbreeding
Pedigree completeness (MacCluer et al., 1983) was computed three (PCI3), five (PCI5) and seven (PCI7) generations back (Figure 3). For the foals born in 2016, PCI3, PCI5 and PCI7 were 1.0, 0.96 and 0.83, respectively. PCI is essentially a measure of the proportion of known ancestral contributions that could contribute to inbreeding. Consequently, low population level PCI will result in underestimation of the level of inbreeding and overestimation of the level of genetic diversity, as unknown relationships are treated as unrelatedness.Giontella, Pieramati, Silvestrelli, and Sarti (2019) reported PCI of 90% and 70% for the third and fifth parental generation of the indigenous Maremmano horse breed, respectively. PCIs for three different reference populations of Hokkaido native horses were reported in Onogi, Shirai, Amano, and T (2017). Estimates for the last reference population (2006–2015) were identical to those for Faroese horses. The pedigree completeness of the tiny pedigree of the Faroese horse is adequate for a realistic estimation of the level of inbreeding.
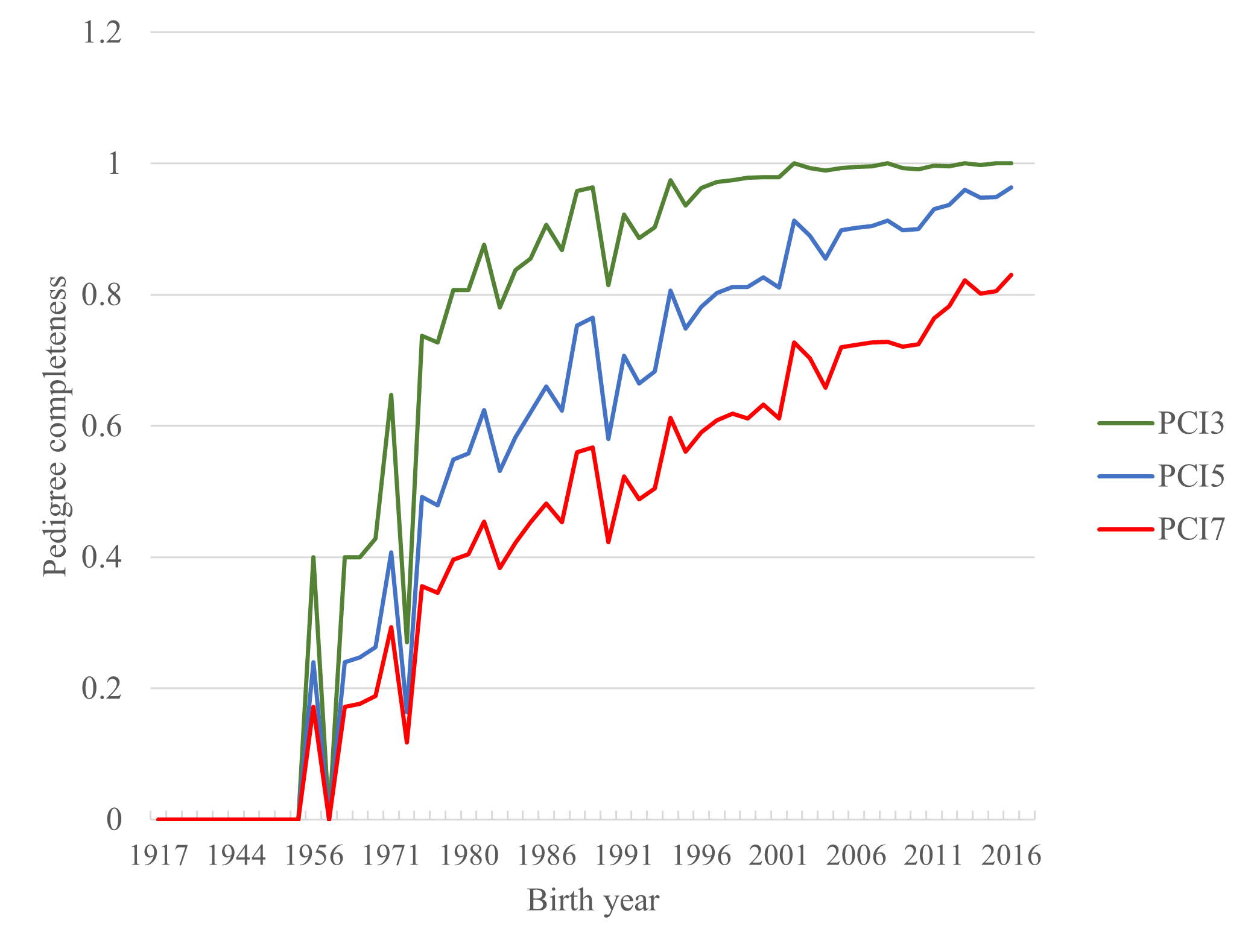
The average co-ancestry and inbreeding in the last cohort in this study (foals born in 2016) were 32.7% and 26.8%, respectively. As the number of breeding animals was very limited, the use of very old individuals for breeding was common. For individuals born in 1980 and onwards the average age of dams was 11.7 years and sires 9.8 years, resulting in an average generation interval of 10.7 years. A total of 22 matings in the whole pedigree were between half-sibs (12.4%), 3 between full-sibs (1.7%) and 15 (8.4%) between parent and offspring. The average inbreeding over birth years fluctuated, and inbreeding in some birth years was governed by one or very few individuals (Figure 4). The individual inbreeding coefficients in the total pedigree (excluding founders and half-founders) varied between 0.8 and 41.3%.
Sire–daughter mating between the same two individuals resulted in highly inbred individuals born in 1956 and 1958. There was a rapid increase in the level of inbreeding during the 1970s. This was a consequence of the bottleneck in the 1960s, when only five individuals were alive. From 1980 onwards, the increase in inbreeding was moderate (Figure 4), with exception of the year 1990 (2004), where only one (two) foal(s) was born with a very high inbreeding coefficient. The overall average inbreeding was 21.9%, with 89.9% inbred animals. A total of 48.2% individuals have inbreeding coefficient corresponding to half-sib to full-sib mating, and 11.3% of the horses had inbreeding coefficients above 30%. Average inbreeding of the Faroese horse is much higher than that reported for different horse breeds in literature; average inbreeding in native horse populations is typically below 10% (Duru, 2017; Gandini, Bagnato, Miglior, & Pagnacco, 1992; Giontella et al., 2019; Mancin et al., 2020; Onogi et al., 2017; Perdomo-González, Sánchez-Guerrero, Molina, & Valera, 2020; Poyato-Bonilla et al., 2020; Sairanen, Nivola, Katila, Virtala, & Ojala, 2009). Slightly higher pedigree-based inbreeding coefficients have been reported for Old Kladruber horse, Warmblood trotter and Gotland Russ (Kvist et al., 2019; Vostrá-Vydrová, Vostrý, Hofmanová, Krupa, & Zavadilová, 2016). Similarly to the Faroese horse population, extremely high inbreeding (32.5%) has been reported in a small population of the endangered Sorraia horse (Luís, Cothran, Mdo, & M, 2007).
Average coancestry among parents of a cohort expresses the expected level of inbreeding of the progeny that results from random mating. The realized inbreeding of the progeny in Faroese horses is slightly lower than the expected inbreeding. This indicates that, despite the high relatedness between available breeding candidates, breeders have mated individuals less related than expected with random mating (Figure 4). This said, the same phenomenon could arise if matings resulting in highly inbred progeny are partly unsuccessful, e.g. due to lethal allele combinations, and consequently fewer offspring would be born from these matings.
Although no apparent signs of inbreeding in the Faroese horse were found in Mikko et al. (2004), it is expected that matings resulting in extremely high inbreeding are not always successful. Adverse effects of inbreeding on reproduction have been documented in literature. Results from Sairanen et al. (2009) confirmed that intense inbreeding as well as the age of both mare and stallion, have an adverse effect on foaling rate. Reproductive problems, such as retained placenta and reduced sperm motility have been reported in Friesian horses and Shetland ponies with inbreeding above 7% and 2%, respectively ( (Onogi et al., 2017) and references therein). Although the population size of Faroese horses has increased during the past three decades, some mares had repeated fertility problems (Joensen, 2019). These mares repeatedly experienced miscarriages, and repeatedly remained empty despite successful mating. A recent study on the Faroese horse concluded that 30% of the mares are infected by equine endometritis, yet infection status was not fully coherent with the fertility status of the mares (Joensen, 2019). The magnitude of inbreeding depression on the Faroese horse reproduction has not been documented. That said, given the generally high level of relatedness of the population, mating of highly related individuals should be avoided.
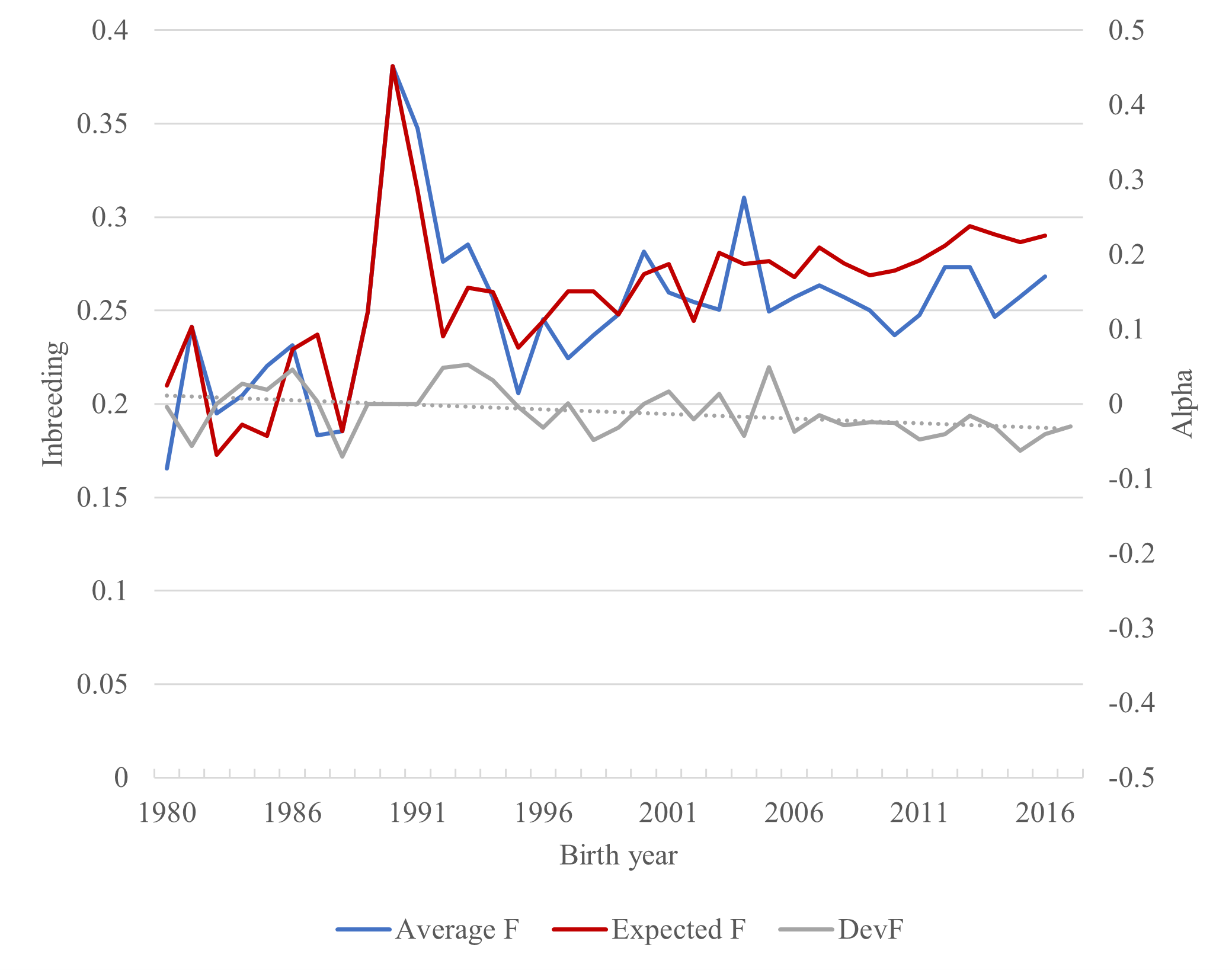
Genetic contributions of founders and ancestors
The base population, defined as the individuals with one or more unknown parents, consisted of ten individuals. The actual base population, where an animal with one unknown parent was defined as a half-founder, consisted of eight animals. The contribution of the ten founder individuals to the last recorded cohort of 2016 varied between 3.0–12.3%. The highest genetic contribution of an ancestor to the most recent cohort was 48.5% from the only living stallion in the 1960s bottleneck (Figure 1). The second-largest genetic contribution of 37.1% was from its son born in 1972. Only one of the mares alive during the 1960s was unrelated, and contributed 24.6% to the 2016 cohort. The three remaining mares alive during the bottleneck were sired by one sire only; its genetic contribution to the latest cohort was 22.3%. The genetic contributions of the dam and its two daughters were 15.2%, 16.3% and 7.0%, respectively. Only two ancestral individuals accounted for 50% of the variation in the population with known parents. The genetic contribution of the ancestors sums up to more than 100% as the genetic contributions in this extremely small pedigree are highly dependent.
Genetic diversity and effective population size
Effective number of founders (fe), ancestors (fa), non-founders (fne) and genomic equivalents (fge) were 9, 3, 1.7 and 1.4, respectively, with reference population of all animals with both parents known. Parameter fe is defined as the number of equally contributing founders that would result in the genetic diversity of the population under study. It only accounts for the loss in genetic variability due to unequal contribution of founders (Lacy, 1989), thus it is not very useful for assessing genetic diversity. Parameter fa is defined as the minimum number of ancestors explaining the complete genetic diversity of the population, and accounts for potential bottlenecks in the pedigree (Boichard et al., 1997). The amount of genetic drift since the foundation of the population is reflected by fne. Finally, fge represents the cumulative loss of genetic diversity since the base population and directly relates to Ne (Caballero et al., 2000; Lacy, 1989; Lacy, 1995).
We used probability of gene origin parameters to derive genetic diversity measures to estimate the degree of genetic diversity relative to the base accounting for loss of diversity due to unequal founder contribution, bottlenecks and genetic drift. The total loss of genetic diversity relative to the base population was 35.8% ( ) (Caballero et al., 2000; Lacy, 1995). Proportionally, 84.5% of this loss could be allocated to bottlenecks and random genetic drift ( ). Consequently, 5.6% of the genetic diversity relative to the base ( ) was lost due to unequal founder contribution (15.5% of the total diversity loss) (Lacy, 1995).
In literature, estimates of parameters of gene origin are frequently used to describe the genetic diversity of horse populations (Duru, 2017; Giontella et al., 2019; Mancin et al., 2020; Onogi et al., 2017; Perdomo-González et al., 2020). Ratios of the parameters have been used to interpret the loss of genetic diversity (Onogi et al., 2017; Perdomo-González et al., 2020), but ignoring the definitions of the quantification of the proportion lost/retained relative to the base (GD, GD*, GD*-GD) in a quantitative manner (Lacy, 1995). This leads to ambiguous interpretations of the parameter ratios as indicators of the origin of the loss of the genetic variation between studies. Following Lacy (1995), the complete loss of genetic variation relative to the base for Pura Raza Español (Perdomo-González et al., 2020), Turkish Arab horse (Duru, 2017), the Italian Maremmano horse (Giontella et al., 2019), the Hokkaido native horse (Onogi et al., 2017) and Italian heavy draught horse (Mancin et al., 2020) is small compared to the Faroese horse: 5.7%, 5.2%, 3.6%, 2.7% and 0.7%, respectively. A slightly higher degree of loss in genetic diversity was estimated for the endangered Old Kladruber horse: 10.5–17%, depending on the reference population (Vostrá-Vydrová et al., 2016). The genetic diversity of the Faroese horse based on the pedigree analysis is extremely low.
Regression coefficients of the individual inbreeding coefficients on the number of i) complete generations (Ia), ii) maximum number of generations (Ib) and ii) equivalent generations (Ic) traced back to founders for each individual were computed. Method Ia and Ic give information on the lower and upper limits of Ne, whereas method Ib should indicate the 'real' Ne (Gutiérrez et al., 2005). The estimates of Ne from the regression-based methods ranged from 8.54 to 24.39 (Table 1). Methods V and VI (Table 1), based on individual increase in inbreeding and coancestry, account for the whole population history estimated Ne to be 8. Estimates of Ne for local breeds such as Turkish Arab, Italian Maremmano, Hungarian Hucul and Italian heavy draught horse estimated with methods V and VI (Table 1 ) have been considerably larger than those for the Faroese horse: 42–97 (Duru, 2017; Giontella et al., 2019; Mancin et al., 2020; Somogyvári, Posta, & Mihók, 2018). Despite the slight decrease in the rate of inbreeding in recent years, all the estimates of Ne for the Faroese horse are critically low, and far from the recommended Ne of 50 to 100 to ensure the sustainable management of this endangered horse breed.
|
|
Method |
N e |
Whole pedigree |
|
|
|
|
Ia: |
Regression on complete generations |
8.54 |
|
Ib: |
Regression on maximum generations |
24.39 |
|
Ic: |
Regression on equivalent generations |
12.69 |
|
II: |
Regression on birth date |
11.04 |
|
IIIa: |
Log regression on birth date |
11.57 |
Restricted pedigree* |
|
|
|
|
IIIb: |
Log regression on equivalent generations |
15.37 |
|
IV**: |
Regression on equivalent generations |
14.16 |
|
V: |
Individual increase in inbreeding |
7.99 (1.35) |
|
VI: |
Individual increase in co-ancestry |
7.88 (0.43) |
Optimum contribution selection
To test whether optimum contribution selection is successful in alleviating problems with inbreeding, we assessed breeding scenarios of 6 to 12 matings, where males were mated with a maximum of 1 to 4 females, relative to the average relationship (Figure 5) and selected individuals (Figure 6).
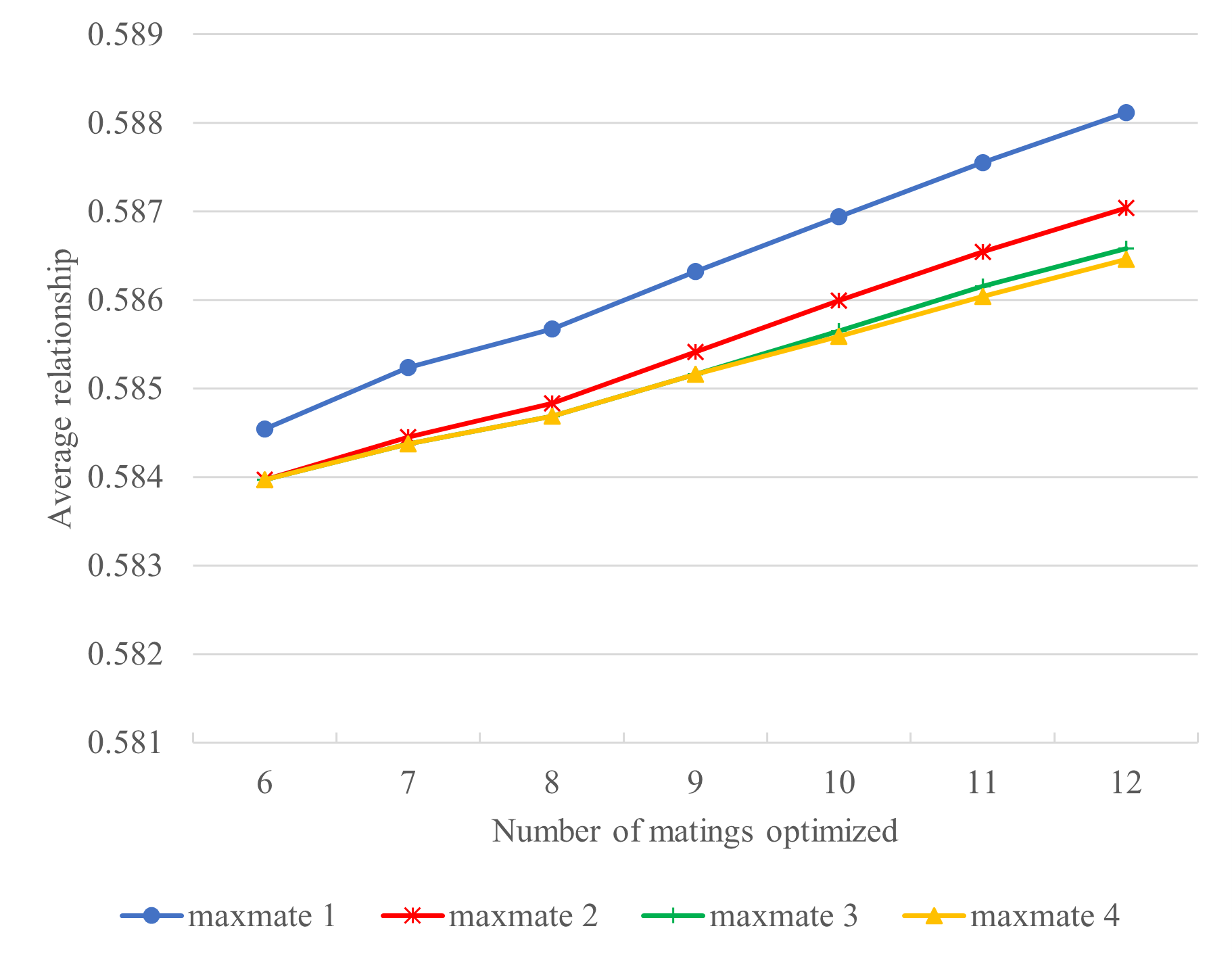
Within a fixed number of matings the repetitive use of stallions resulted in the lowest level of average relationships, although the differences between scenarios were very small and at maximum approximately 0.4% unit (Figure 5, maxmate = 1, 6 vs 12 matings). In an extremely small and inbred population, as is the case of the Faroese horse, an increase in the number of selected individuals inevitably results in the selection of more related individuals.
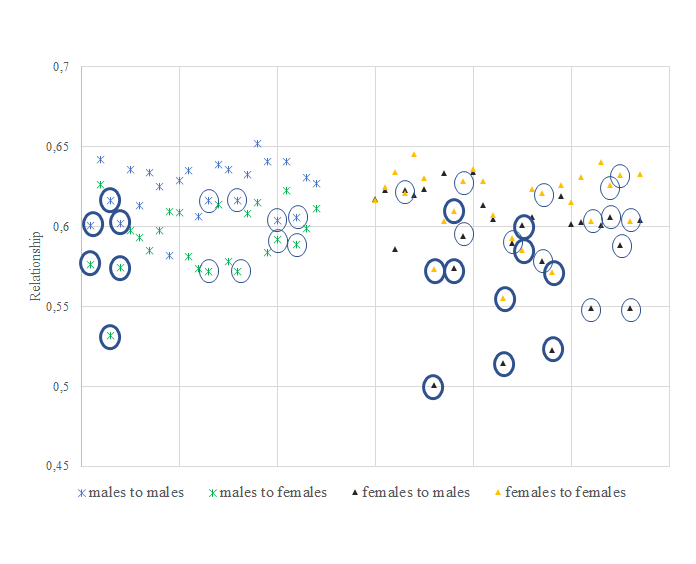
A minimum of four and a maximum of seven stallions were selected for breeding over all the scenarios allowing repetitive use of stallions. A subset of the same three stallions was selected in all scenarios, and in total only seven out of the 24 available stallions were ever selected across all scenarios (Figure 6). The additive genetic relationship of each of the selected males with all candidate males (0.60–0.62) and females (0.53–0.59) was equal to or lower than the average amongst male candidates (0.62) or between male and female candidates (0.59). Additionally, the additive genetic relationship of the selected males with the individuals born during the last two years (2015–2016) was lower than the average of all candidate males: 0.57 vs. 0.60. Similarly, the additive genetic relationship of the selected females with all candidate females (0.60) and males (0.57) was lower than that amongst female candidates (0.62) or between female and male candidates (0.59). The additive genetic relationship of the selected females with individuals in the last two cohorts was lower than that of all female candidates: 0.58 vs. 0.60.
The potential of OCS for optimization of genetic gain in performance and increase in relatedness in e.g. the Franches‐Montagnes horse, Menorca horse and Norwegian and North-Swedish trotter has been assessed by Hasler et al. (2011), Olsen, Meuwissen, and Klemetsdal (2013) and Solé, Valera, Gómez, Cervantes, and Fernández (2013). Similar to the Faroese horse, full weight on average relationships and minimization of inbreeding when optimizing matings for the endangered Jutland horse were used in Nielsen et al. (2020); authors reported that preselection of sires and OCS successfully lowered the average inbreeding compared to random mating. Sustainable breeding of an extremely small and a priori highly inbred population is of great importance. Successful follow-up of mating schemes planned with OCS helps to avoid a rapid increase in inbreeding in future generations, as well as minimizes inbreeding of single matings. Therefore, whether an individual is eligible as a breeding candidate should be evaluated thoroughly relative to the health of the individual and possible previous experiences regarding fertility and mothering characters. This is to avoid that the planned mating will not be realized or does not result in viable progeny. The Felagið Føroysk Ross has adopted the use of a database, Førøya Fongur, to collect all information (e.g. pedigree, health, mating, progeny) on the Faroese horse population. Information collected in the database on reproduction and health will assist in the recognition of possible inbreeding depression, but this process is still in its infancy. The Government of the Faroe Islands provides support (currently managed by the Agricultural Agency of the Faroe Islands) to horse owners if their horses are registered in the database and are producing offspring. Unfortunately, the breeder can receive support even if the planned mating would not be appropriate relative to the resulting inbreeding of the offspring. Overall, the maximization of reproductive success is of high priority to be able to expand the census size.
Conclusions and recommendations
Results from this study are in accordance with the documented population history of the Faroese horse; the population has extremely high average relatedness and inbreeding, and low effective population size. Consequently, the census size should be expanded as it sets the limit of constraining the future rate of inbreeding. It is recommended to optimize the contributions of parents to the next cohort to minimize increases in average coancestry of the population. Due to the expected inbreeding depression, the fitness-related traits should be recorded in the database and carefully monitored. To increase the interest in the breed, actions to improve the economic value of the Faroese horse, e.g. through export and increased use in tourism should be promoted. Molecular genetic characterization is recommended to be able to assess the additive genetic relationships between individuals accurately and to heighten the potential of OCS in conserving the Faroese horse.
Acknowledgements
We thank the Felagið Føroysk Ross for providing the data.
Supplemental data
Author contributions
Signa Kallsoy Joensen compiled and quality checked the pedigree data. Anne Kettunen analyzed the data, interpreted the results and drafted the manuscript. Peer Berg wrote EVA. All authors read, revised and approved the final manuscript.
Data availability statement
Anonymized pedigree data is available upon request from the Felagið Føroysk Ross.
Conflict of interest
The authors declare no conflicts of interest.