Evaluating agromorphological traits of Greek wheat landraces and exploring their potential for bread and pasta making based on seed physical properties
Abstract
Physical and morphological characteristics of Greek wheat landrace and cultivar seeds were evaluated, aiming to assess their bread- and pasta-making quality. Furthermore, the plant agromorphological traits of wheat landraces and cultivars were measured and correlated with seed physical properties. Fifteen Triticum spp. accessions, out of which four cultivars (two Triticum aestivum and two T. durum), nine landraces (two T. durum, five T. aestivum, one T. dicoccum and one T. polonicum) and two T. durum of unknown status were studied. Seventeen morphological plant and seed traits were measured based on UPOV descriptors. Ear emergence was earlier in cultivars than in landraces. The plant weight of the landraces was, in some cases, almost twice the cultivars’. Seed firmness (62.96–194.85N) was positively correlated with thousand kernel weight and volume (0.840 and 0.791, P < 0.05, respectively). Based on the agromorphological traits, cluster analysis clearly separated the species and the cultivars from the landraces, and the unknown status accessions were grouped with the cultivars. Cluster analysis with all measured traits set the wheat accessions in the following distinct groups: (1) T. durum cultivars including the unknown status accessions, (2) T. aestivum cultivars, and (3) T. aestivum and T. durum landraces. T. polonicum and T. dicoccum each formed a separate group. Seed physical properties of the analyzed Greek landraces indicated their suitability for bread and/or pasta making.
Keywords
Agromorphological characteristics, image analysis, seed firmness, wheat landraces, seed physical properties, pasta and bread making, bread wheat, durum wheatIntroduction
Many researchers in the past have tried to define the meaning of a landrace, which is also known as “a local, farmer’s, traditional variety or a local population” (Zeven, 1998). Recently, a more general definition for landrace was suggested: “a cultivated, genetically heterogeneous variety that has evolved in a certain ecogeographical area and is therefore adapted to the edaphic and climatic conditions and to its traditional management and uses” (Casañas, Simó, Casals, & Prohens, 2017).
Landraces have been the main crop genetic resources used since the emergence of agriculture (Purugganan, 2019). In the last century, with agricultural intensification, several landraces have been replaced with high-yielding cultivars (hereafter the term cultivar means scientifically improved cultivated variety). The main differences between landraces and cultivars are that the latter are the results of formal breeding research (when compared to farmers’ mass selection for landraces) and they are provided to farmers and growers through formal seed systems (when compared to traditionally informal channels, such as exchanges and local markets for landraces). Landrace cultivation has been almost abandoned and many landraces have been lost, leading to severe genetic erosion (Robbana et al., 2021), which is the loss of crop variation due to the modernization of agriculture (Wouw, Kik, Hintum, Treuren, & Visser, 2010).
In the case of wheat, a study conducted by Haudry et al. (2007), concluded that the estimated initial biodiversity decreased by 84% for durum wheat and by 69% for bread wheat, although such estimations could be controversial (Khoury et al., 2022). Genetic erosion of Triticum aestivum landraces in Ethiopia was found to be 66.7% in 2016 (Gelelcha, Kumsa, & Kuma, 2023).
In Greece, only 3% of the total land surface cultivated with durum wheat was planted with landraces in 1984, and 1.3% in 1991 (Kyzeridis, Biesantz, & Limberg, 1995). On an expedition encompassing 36 villages on Lefkada island, from 2010 to 2012, researchers were able to collect only three wheat accessions, two of which were landraces (Thomas, Thanopoulos, Knüpffer, & Bebeli, 2013). Although the genetic erosion of wheat landraces was shown by collecting expeditions carried out in Greece, they can still be found cultivated on farms (Thanopoulos, Chatzigeorgiou, Argyropoulou, Kostouros, & Bebeli, 2021). The study of landrace properties is crucial both for local communities, which would benefit from the added value of their products, and their use in breeding programmes. Thanopoulos et al. (2021), who collected landraces in Arcadia, Greece, estimated wheat genetic erosion between 1942 and 2021 to be 45.7%. Moreover, recent research revealed a 97% genetic erosion of winter wheat for the years 1955–2015 in Central Europe (Cseh et al., 2021).
Cereal cultivars are high-yielding, but the majority of them is less resilient to abiotic and biotic stresses compared to landraces (Newton et al., 2010). Reduced adaptation to organic, low-input production farming systems and poorer genetic diversity were observed in Tunisian improved durum wheat cultivars, in contrast to landraces (Ayadi et al., 2020). Although further research is required, there is also evidence that ancient wheat types, such as emmer wheat, provide health benefits compared to modern wheat cultivars (Shewry, 2018) and could be a potential source of antioxidants, carotenoids and phenolics (Newton et al., 2010). For such ancient species, like T. dicoccum, and neglected and underutilized species, like T. polonicum, (Bieńkowska, Suchowilska, & Wiwart, 2020; Carvalho et al., 2019) more research to investigate their properties is needed.
Currently, climate change, extensive loss of genetic diversity, increased vulnerability of cultivars to abiotic and biotic stresses and consumer awareness of the value of local products, have raised interest in wheat landraces (Brush, 1995; Moghaddam, Ehdaie, & Waines, 1997; Saleh, 2020; Tomás, Coelho, Rodrigues, Viegas, & Silva, 2020). Wheat landraces could play a crucial role in mitigating the negative effects of climate change due to their ability to perform sufficiently under high temperatures (Ulukan, 2021) and exhibit higher yield stability under water stress (Karamanos, Economou, Sotirakoglou, Lyra, & Papastavrou, 2017). Recent research focused on the collection of landraces, their traits and cultivation revival as well as the promotion of sustainable agricultural production (Newton et al., 2010). It also highlighted the characteristics associated with their utilization in the production of high-quality products for consumers (López-Fernández et al., 2021; Ruisi et al., 2021).
The analysis of the agronomic performance of wheat landraces revealed significant differences in plant height, seed colour and biological cycle (Gharib et al., 2021). A study of morphological characteristics of old Sicilian landraces showed high variability among durum wheat accessions (Sciacca et al., 2014). Similar results were also obtained for days to emergence, tillering, booting, flowering and physiological maturity, plant height, and thousand kernel weight (TKW) in 23 Moroccan durum wheat accessions (Zarkti, Ouabbou, Hilali, & Udupa, 2010). In a study of 63 durum wheat landraces, grain weight was the most significant criterion for improving their adaptation to the northern parts of the Mediterranean basin (Moragues, Moral, Del, Moralejo, & Royo, 2006).
Several studies compared grain characteristics of landraces and other variety types. In one study, ten obsolete varieties (varieties that were developed by systematic breeding efforts in the past and now have been replaced by newer and better-performing cultivars) (Maxted, Kell, & Brehm, 2011) and two wheat landraces from Bosnia and Herzegovina were evaluated for grain length, width, thickness, volume, surface area, TKW and protein content, and most of the traits presented significant interactions among them. Obsolete wheat genotypes ‘Brkulja’ and ‘Šiđanka’ showed the most promising grain characteristics (Kondić, Žuljević, Hajder, & Selimbegović, 2020). Kernel morphology is important for manufacturing final food products, which requires specific grain characteristics (Dholakia et al., 2008). Machine-vision techniques (image analysis) were used as a useful tool for the classification of varieties based on morphology, colour and seed texture of different species (Chaugule & Mali, 2016; Smykalova, Grillo, Bjelkova, Hybl, & Venora, 2011; Smykalova, Grillo, Bjelkova, Pavelek, & Venora, 2013) and wheat seed quality assessment (Venora, Grillo, & Saccone, 2009). Other studies evaluated quality parameters, such as starch and protein content of wheat flour obtained from landraces in comparison to cultivars. Boukid et al. (2018) detected higher values of protein and gliadin in Tunisian durum wheat landraces, while modern genotypes had significantly higher values of total starch.Zheng, Sun, and Pan (2012) studied 477 Chinese bread wheat landraces (T. aestivum L.) and concluded that there was a significant correlation between starch and protein content. Mediterranean durum wheat landraces presented a higher amount of protein content but lower gluten strength than cultivars (Roselló et al., 2018). In addition, a lower content of water-extractable arabinoxylans, the main non-starch polysaccharides in cereals, was found in Turkish landraces (T. monococcum, T. durum and T. aestivum) than in cultivars (T. aestivum) (Cetiner, Tömösközi, Török, Salantur, & Koksel, 2020). The comparison of different aromatic profiles and the characteristics of the processed products showed high diversity in Sicilian durum wheat landraces in contrast to the uniformity of cultivars (Ruisi et al., 2021). Recently, some studies focused on the physical or functional characteristics of final products made from wheat landraces. For example, some soft wheat landraces could be used for biscuit production, since their grain hardness did not significantly reduce biscuit quality (Igrejas, Guedes-Pinto, Carnide, Clement, & Branlard, 2002). Spanish landraces showed lower bread-making quality properties because of their low-quality protein compared to cultivars (Gómez, Aparicio, Ruiz-París, Oliete, & Caballero, 2009). High variability of Mediterranean landraces could be used for improving gluten quality (Nazco et al., 2014).
Even though many papers separately examine either plant agromorphological traits or seed physico-mechanical properties, there is limited published work that studies holistically the above traits with both agronomic and food technology approaches. For instance, in a comparative study of five cultivars and two landraces, the latter were significantly taller, flowered later, and had lower yields and gluten index but higher protein content in comparison to wheat cultivars (Preiti et al., 2022). This field needs more data to critically evaluate this issue. The purpose of the present study was to characterize wheat landraces and cultivars according to morphological, agronomical, seed quality and technological traits and investigate their potential for bread and/or pasta making. Specifically, the research aimed to: (1) study the agromorphological characteristics and seed physical properties, (2) compare T. durum, T. aestivum, T. dicoccum, and T. polonicum wheat landraces with cultivars, (3) classify all the accessions (cultivars and landraces) based on agromorphological traits, separately or on the whole set of traits (both agromorphological and seed physical properties) and (4) identify the wheat landraces more suitable for bread- and pasta-making purposes.
Materials and methods
Plant material
The plant material consisted of 15 Triticum spp. accessions, namely two cultivars and five landraces of T. aestivum, two cultivars, two landraces and two accessions of unknown status of T. durum, one landrace of T. polonicum and one landrace of T. dicoccum. The latter was collected in the early 20th century in Greece, it was conserved in USDA-GRIN (Code PI 94682) and repatriated recently. Landraces were collected from different regions in Greece and donated to the Plant Breeding and Biometry Laboratory of the Agricultural University of Athens (AUA) (Table 1 and Figure 1). The Institute of Plant Breeding and Genetic Resources (IPGRB) donated four cultivars, recommended for bread making (‘Yekora’ and ‘Elisavet’ belonging to T. aestivum) and pasta making (‘Mexicali 81’ and ‘Elpida’, of T. durum) which were used as controls.
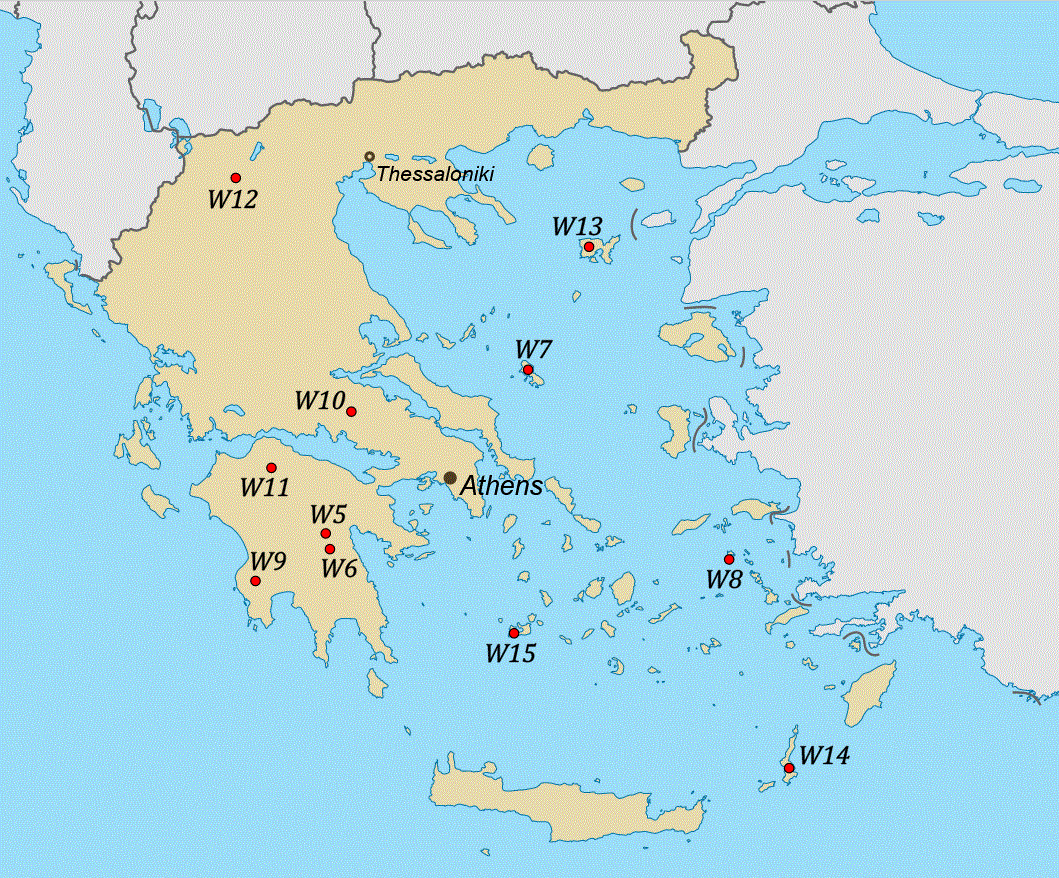
Accession code |
Name |
Triticum species 1 |
Breeding status |
Origin |
Collection date |
W1 |
Yekora |
T. aestivum L. |
Cultivar |
IPGRB3 |
Donated 2019 |
W2 |
Elisavet |
T. aestivum L. |
Cultivar |
IPGRB |
Donated 2019 |
W3 |
Elpida |
T. durum Desf. |
Cultivar |
IPGRB |
Donated 2019 |
W4 |
Mexicali 81 |
T. durum Desf. |
Cultivar |
IPGRB |
Donated 2019 |
W5 |
Zoulitsa |
T. aestivum L. |
Landrace |
Arcadia-Peloponnese |
2018 |
W6 |
Ntopio |
T. aestivum L. |
Landrace |
Arcadia-Peloponnese |
2018 |
W7 |
Mavragani- Skyros |
T. durum Desf. |
Unknown status |
Skyros Island |
2012 |
W8 |
Grilos |
T. aestivum L. |
Landrace |
Patmos Island |
2013 |
W9 |
Leventis |
T. polonicum L. |
Landrace |
Messinia-Peloponnese |
2013 |
W10 |
Kopaida |
T. dicoccum Schrank |
Landrace |
USDA-GRIN, Central Greece |
Repatriation 2013 |
W11 |
Asprositi -Kalavrita |
T. aestivum L. |
Landrace |
Kalavrita-Peloponnese |
2019 |
W12 |
Asprositi - Kozani |
T. durum Desf. |
Landrace |
Kozani-West Makedonia |
2005 |
W13 |
Mavragani- Lemnos |
T. durum Desf. |
Unknown status |
Lemnos Island |
2019 |
W14 |
Aspratheri |
T. durum Desf.2 |
Landrace |
Karpathos Island |
2019 |
W15 |
Kokkinositaro |
T. aestivum L. |
Landrace |
Milos Island |
2019 |
Experimental design
The experiment was conducted at an experimental field of the Agricultural University of Athens (location: 37° 59' 06.8" N, 23° 42' 24.7" E, altitude 24m a.s.l.) during the winter growing season of 2019–2020. The meteorological data are presented in Figure 2. The values of mean air temperature and precipitation for the Athens region during the years 2015–2020 were provided by the Hellenic National Meteorological Service (HNMS, 2023). A randomized complete block design with three replications was used. Each replication consisted of 20 plants of each accession sown in rows spaced 0.2m apart. Sowing distances among plants in each row was 20cm. The sowing date was mid-November, a typical period for sowing in central Greece.
Manipulation of sowing time is one of the most effective techniques for the production of high-quality wheat kernels (Butkovskaya & Kozulina, 2021). In the present study, for comparison reasons, plant sowing was carried out simultaneously for all accessions, without considering the best sowing time for each accession.
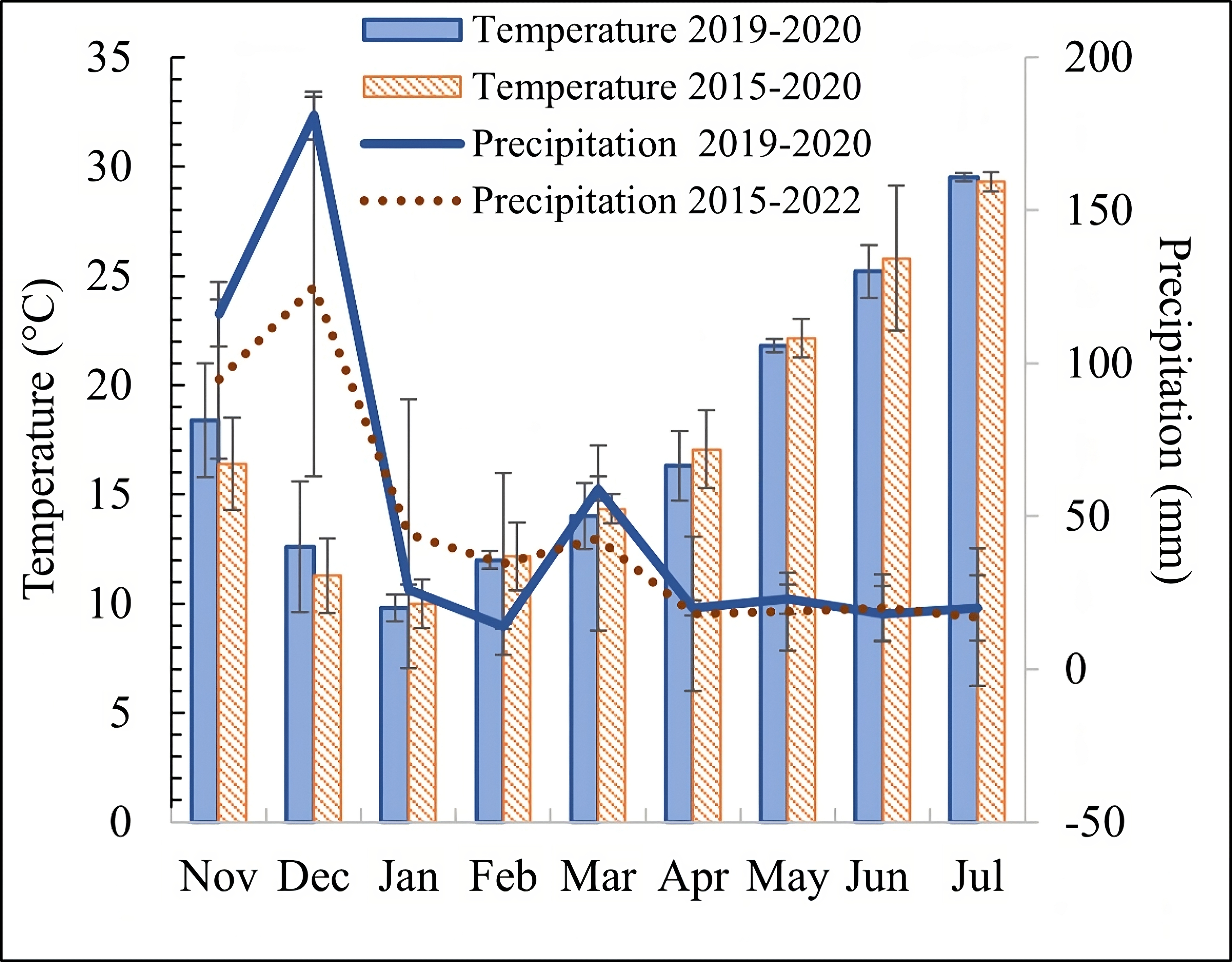
Measurement of agromorphological traits
During vegetative and reproductive stages, time of ear emergence (days), total number of tillers, ear length excluding awns (cm), ear length including awns (cm), number of spikelets in the spike of the first tiller, plant length (measured from the base of the plant to the tip of the highest awn, cm), stem length (measured from plant base to ear base, cm) and whole plant weight (physically dried by sun, in g/plant) were measured. In addition, the qualitative variables ear colour, ear shape in profile, growth habit and awn colour, were scored. Agromorphological traits were evaluated on all the plants per plot to calculate the mean value for the quantitative traits and the median for the qualitative traits. The measurements of the following traits: plant length, growth habit, ear emergence, ear length, ear colour, ear shape, and awn colour were carried out using UPOV descriptors (UPOV, 2012; UPOV, 2017).
Physical properties of seeds
Before measurements, shrunken, broken kernels, and other impurities were removed. The weight of 1,000 randomly selected kernels of wheat was measured for thousand kernel weight (TKW). The same 1,000 selected kernels were then filled into a volumetric cylinder to determine their volume. Tap volume was measured after gently tapping the cylinder 100 times or until there was no further decrease in the sample level. Bulk density (ρb) and tap density (ρt) were calculated as weight of sample per volume and per tap volume of sample, respectively (g/ml) (Chaloulos, Bazanis, Georgiadou, Protonotariou, & Mandala, 2021).
Determination of flow properties is essential in processes such as transportation, mixing and storage (Fitzpatrick, Barringer, & Iqbal, 2004). The Carr index (CI) was used to calculate the flowability of the seeds (Carr, 1965):
CI (%) = (𝜌𝑡 − 𝜌𝑏) ⁄𝜌𝑡 × 100
The firmness of seed (SF), defined as the force required to crush the seed, was estimated using an Instron (Universal Testing Machine, Model 3343, Nordwood, MA, USA) equipped with a 1kN load cell. Fifty intact seeds of each accession were compressed to 50% of their initial width with a 4cm diameter probe and a speed of 1mm/min.
Seed colour was measured using the colourimeters 3nh High-Quality Spectrophotometer NS800S (Shenzhen 3nh Technology, China), according to CIE-L*a*b* uniform colour space, where L* indicates lightness, a* indicates hue on a green (−) to red (+) axis, and b* indicates hue on a blue (−) to yellow (+) axis. White index (WI) was also calculated according to the following equation:
Image analysis-shape factor measurements
One hundred seeds of each accession were scanned twice using a flatbed scanner (HP scan jet 4370, Hewlett Packard, USA). Images of the seeds were captured for further analysis using an image analysis software (Image Pro Plus 7, Media Cybernetics, USA). An indicative image of the scanned seeds is presented in Figure 3A. Area (mm2), aspect, average optical density (density/intensity mean), mean, maximum and minimum diameter (mm), perimeter (mm), roundness, size length (mm) and width (mm) were determined. Detailed descriptions and definitions of shape factors are presented in Figure 3B. The measurements were done in triplicates.
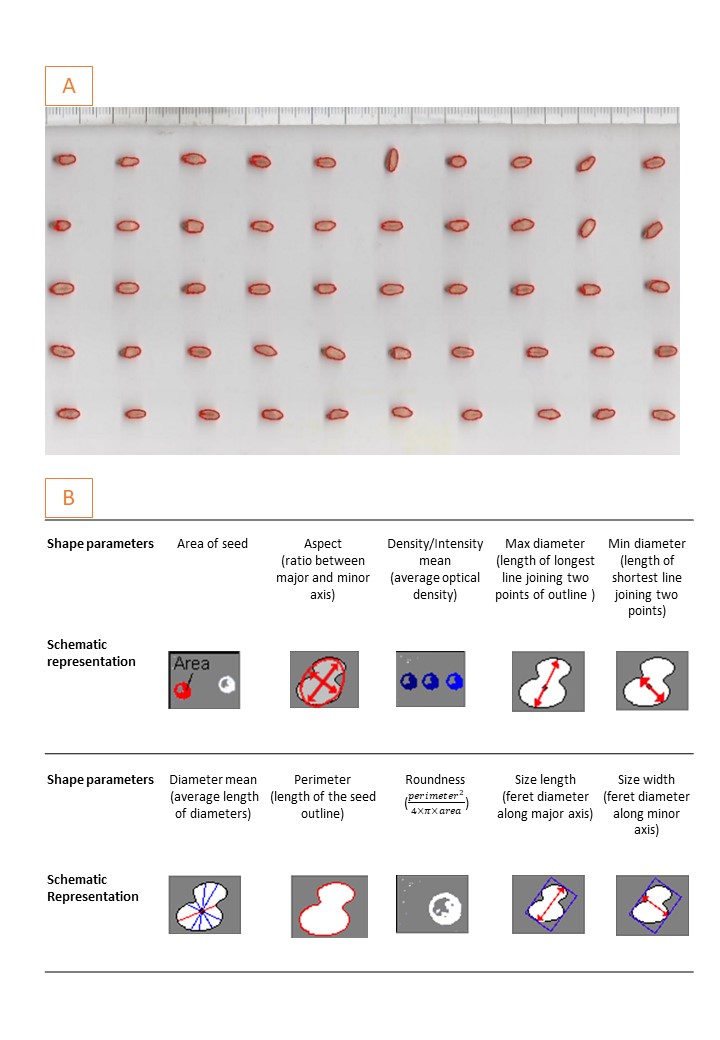
Statistical analysis
Statistical analysis of the data was performed with Statgraphics Centurion XV (Statgraphics, Rockville, MD, USA). For qualitative variables (plant growth habit, colour of ear, ear shape in profile and awn colour) frequency percentages (Supplemental Figure 1) and median values were calculated (Supplemental Table 1). Friedman Test was used to test the null hypothesis that the mean ranks of the samples groups are the same (Supplemental Table 1).
Quantitative trait data were subjected to analysis of variance after testing the assumptions of normality and homoscedasticity with Levene's and Bartlett's tests respectively (Supplemental Table 2). The comparisons of the means were performed using the Tukey HSD criteria with a level of significance of α = 0.05.
The correlation between quantitative parameters was assessed by Pearson's correlation test (significance level at α = 0.05). The correlation between qualitative parameters (nonparametric measures) was assessed by Spearman rank correlation test (significance level at α = 0.05). To correlate quantitative and qualitative variables the Spearman test was also used (Supplemental Table 3). Cluster analysis was used to group the quantitative observations with similar characteristics using nearest neighbour (single linkage) and distance metric of squared Euclidean on standardized data.
Results and discussion
Agromorphological traits
Mean values of quantitative agromorphological traits for all studied wheat accessions are presented in Table 2. Time of ear emergence ranged from 107 to 138 days (Yekora - T. aestivum, cultivar, and Zoulitsa - T. aestivum, landrace, respectively). All cultivars, except Elisavet - T. aestivum, were earlier than landraces. Similarly,Frankin et al. (2021) reported cultivars to be on average 12% earlier than landraces. On the other hand, the landraces Zoulitsa (T. aestivum), Kopaida (T. dicoccum) and Leventis (T. polonicum) had the latest heading time of all accessions. The T. aestivum landraces, Asprositi Kozani (W12), Ntopio (W6) and Asprositi Kalavrita (W11) exhibited early maturation compared to other landraces. Consequently, these landraces have the potential to be incorporated into breeding programmes focused on selecting for early maturation traits (Javaid, Rabbani, & Rastina, 2005). Time of ear emergence was positively correlated with total number of tillers, plant length (from the base of the plant to the tip of the highest awn) and stem length (0.584, 0.674 and 0.710, P < 0.05, respectively) (Table 3). A positive correlation between days of heading and number of fertile tillers was also recorded in a study with 64 bread wheat genotypes in West Shewa and found that days to heading were positively correlated with the number of productive tillers (Mecha, Alamerew, Assefa, Assefa, & Dutamo, 2017). In the study of Bilgrami et al. (2020), where bread wheats were used, the total tiller number was also significantly correlated with days from sowing to heading (0.22, P < 0.05). Although landraces had more tillers than cultivars, the difference between landraces and specific cultivars was not statistically significant and the number of tillers ranged from 4.18 (Mavragani Lemnos - T. durum, unknown status, W13) to 10.38 (Grilos - T. aestivum, landrace, W8).
Accession code and name |
Time of ear emergence (days) |
Plant weigth (g/plant) |
Total no. of tillers |
Ear length excluding awns (cm) |
Ear length including awns (cm) |
No. of spikelets in the first tiller |
Stem length (cm) |
Plant length (cm) |
W1, Yekora |
107.00a |
17.55a |
7.05abcde |
10.24cde |
15.53abc |
17.56abcd |
68.35a |
83.88a |
W2, Elisavet |
115.67bcd |
21.11a |
5.91abc |
11.18de |
14.94 ab |
20.18de |
73.62a |
88.56a |
W3, Elpida |
107.00a |
25.33ab |
6.96abcde |
7.33ab |
17.35 abc |
14.90a |
88.68b |
106.03abc |
W4, Mexicali |
108.00a |
25.02ab |
6.49abcd |
7.14ab |
17.97abc |
15.53ab |
85.90b |
103.87abc |
W5, Zoulitsa |
138.00h |
35.70abcd |
8.64cde |
10.97cde |
18.54abc |
15.57abc |
122.06ef |
140.60defg |
W6, Ntopio |
121.50ef |
47.14bcd |
8.28bcde |
12.27e |
20.16abc |
17.88abcd |
137.17 |
158.25fg |
W7, Mavragani Skyros |
110.00ab |
24.42ab |
5.18ab |
6.53a |
18.04abc |
15.80abc |
75.14a |
93.18ab |
W8, Grilos |
121.33def |
39.17abcd |
10.38e |
11.44de |
18.01abc |
16.57abc |
110.41cd |
127.26cde |
W9, Leventis |
128.67g |
58.30de |
7.11abcde |
10.69cde |
23.81cd |
19.26cde |
130.12fg |
153.97efg |
W10, Kopaida |
135.00h |
31.22abc |
10.28e |
7.57ab |
18.20abc |
19.00bcde |
101.62c |
118.00bcd |
W11, Asprositi Kalavrita |
123.33fg |
28.97abc |
7.01abcde |
12.11e |
12.67a |
16.31abc |
118.17de |
125.15cde |
W12, Asprositi Kozani |
117.50cde |
73.01e |
9.74de |
9.40bcd |
22.16bcd |
21.81e |
118.33de |
144.36cdef |
W13, Mavragani Lemnos |
112.67abc |
20.42a |
4.18a |
6.52a |
18.36abc |
15.97abc |
71.12a |
87.07a |
W14, Aspratheri |
123.67fg |
50.82cde |
8.73cde |
8.41abc |
21.77bcd |
20.22de |
137.27g |
159.04g |
W15, Kokkinositaro |
123.33fg |
32.68abc |
8.06bcde |
11.58de |
29.17d |
17.65abcd |
123.11ef |
152.28efg |
In most cases, the landraces had higher total plant weight than the cultivars. The weight of the landraces ranged from 28.97g/plant (Asprositi Kalavrita - T. aestivum, landrace, W11) to 73.01g/plant (Asprositi Kozani - T. durum, landrace, W12), while cultivars’ weight ranged from 17.55g/plant (Yekora - T. aestivum, cultivar, W1) to 25.33g/plant (Elpida - T. durum, cultivar, W3). This could be attributed to the higher biomass produced by landraces, a characteristic desirable by farmers who need straw for their animals (Thanopoulos et al., 2021). Plant weight was positively and moderately correlated with the total number of tillers and ear length including awns (0.570 and 0.526, P < 0.05, respectively) and highly correlated with the number of spikelets, plant length and stem length (0.644, 0.796 and 0.759, P < 0.01, respectively). The number of spikelets per spike of the first tiller did not differ significantly between accessions.
|
Time of ear emergence (days) |
Plant weight (g) |
Total no. |
No. of spikelets (first tiller) |
Plant length (cm) |
Stem length (base to ear base) (cm) |
Ear length excluding awns (cm) |
Plant weight |
0.404 (0.135) |
|
|
|
|
|
|
Total no. of |
0.584 (0.022) |
0.570 (0.026) |
|
|
|
|
|
No. of spikelets |
0.256 (0.358) |
0.644 (0.010) |
0.392 (0.148) |
|
|
|
|
Plant length |
0.674 (0.006) |
0.796 (0.000) |
0.608 (0.016) |
0.375 (0.168) |
|
|
|
Stem length (base to ear base) (cm) |
0.710 (0.003) |
0.759 (0.001) |
0.611 (0.016) |
0.330 (0.230) |
0.988 (0.000) |
|
|
Ear length excluding awns (cm) |
0.409 (0.131) |
0.242 (0.385) |
0.344 (0.209) |
0.207 (0.459) |
0.456 (0.088) |
0.499 (0.058) |
|
Ear length including awns (cm) |
0.267 (0.337) |
0.526 ( 0.044) |
0.267 (0.337) |
0.325 (0.238) |
0.64 3 (0.010) |
0.523 (0.045) |
0.042 (0.883) |
Cultivars were significantly shorter than the landraces, which is due to the introduction of Rht (Reduced height) genes in modern wheat varieties. These dwarfing genes have been used in wheat breeding to develop cultivars with short stature (Acquaah, 2012). Similar results were recorded in another study, where Italian landraces were found to have taller culms compared to cultivars (Preiti et al., 2022). The plant length for the cultivars was between 83.88cm (Yekora - T. aestivum, cultivar, W1) and 103.87cm (Mexicali 81 - T. durum, cultivar, W4), while for the landraces the plant length was between 118cm (Kopaida - T. dicoccum, landrace, W10) and 159.04cm (Aspratheri - T. durum landrace, W14). Mavragani accessions, W7 and W13, of unknown breeding status, were short, with 87.07cm and 93.18cm, respectively. These values did not differ significantly from cultivar plant length (W1 - W4) (Table 2). Low plant height is a desirable trait for commercial varieties, as it is correlated with good standing ability and resistance to lodging (Würschum, Langer, Longin, Tucker, & Leiser, 2017). The weak point of wheat landraces is the lodging, but under climatic change and high temperatures, taller plants could demonstrate higher yield than short ones (Jatayev et al., 2020).
Plant growth habit was significantly correlated with weight per plant, total number of tillers, plant length and stem length (base to ear) (0.525, 0.623, 0.538, 0.529, respectively P < 0.05) (Supplemental Table 3). A higher number of tillers leads to an increased inclination of the plant.
Cluster analysis based on the quantitative agromorphological traits grouped all cultivars with two Mavragani accessions of unknown status (W7 and W13) in cluster 1, while all landraces were included in a distinct cluster (cluster 2) (Figure 4). The similarity of Mavragani durum wheat accessions from Skyros and Lemnos, with wheat cultivars indicates that these accessions could be old cultivars grown and reproduced for years by farmers, who considered them of local origin (Thomas et al., 2013). Overall, the quantitative agromorphological traits based on UPOV descriptors were sufficient to separate wheat accessions by their breeding status, namely landraces or cultivars.
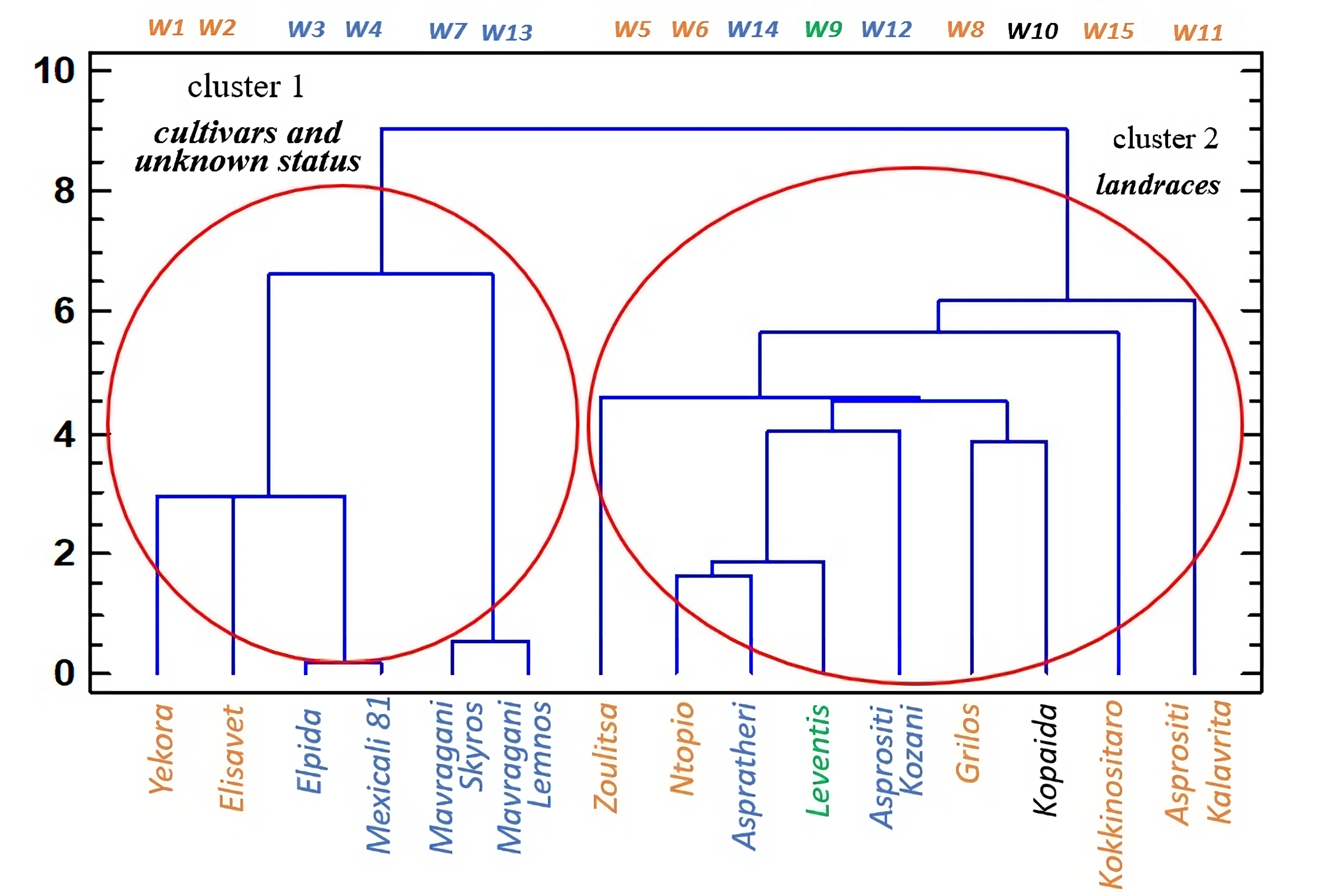
Physical properties of seeds
TKW is of great interest (Javaid et al., 2005) as it is an important index for the prediction of flour extraction rate (Posner, 2009). Higher values of TKW indicate a higher percentage of endosperm leading to higher flour yield (Wiersma, Busch, Fulcher, & Hareland, 2001). TKW values of the studied accessions are presented in Figure 5. TKW of W9 (T. polonicum) was significantly higher (80.45g) than all other accessions. W10 (T. dicoccum) presented the lowest value of TKW (26.92g), consistent with previous research, where the TKW of 38 emmer wheat accessions, collected in several European countries, ranged from 22.9g to 42.6g (Mondini, Grausgruber, & Pagnotta, 2013). T. dicoccum presented significantly lower values of TKW compared to T. durum, 32.02–36.12g and 45.17–46.31g, respectively (Shoormij, Mirlohi, Saeidi, & Mohammadi, 2022). TKW in the rest of the accessions, either cultivars or landraces, ranged from 34.08g (Elisavet - T. aestivum, cultivar, W2) to 67.16g (Mavragani Lemnos - T. durum, unknown status, W13) and were in accordance with Travlos, Karamanos, Economou, and Papastavrou (2012) who studied five Greek bread wheat landraces (30–50.7g). Specifically, in the present study, W5 (Zoulitsa) had an average TKW of 43.66g, while in Travlos et al. (2012), TKW in Zoulitsa ranged from 36g to 44g, indicating that climatic conditions can affect TKW but also the origin of the genetic material (Papadakis, 1929). Moreover, it is worth noting that in the present study, T. aestivum in most cases presented significantly lower values of TKW compared to T. durum accessions. Landraces had similar or higher values of TKW compared to cultivars of the same species, indicating that landraces could give high flour yield. However, results from previous research are contrasting as no significant difference between landraces’ and cultivars’ TKW was reported for Turkish (Cetiner et al., 2020) and Sicilian wheats (Ruisi et al., 2021). On the contrary, Spanish bread wheat landraces showed higher TKW compared to a reference set (López-Fernández et al., 2021). In another research, similar TKW values for T. aestivum landraces and cultivars were detected, while T. durum cultivars, compared to landraces, had significantly higher TKW values (Frankin et al., 2021).
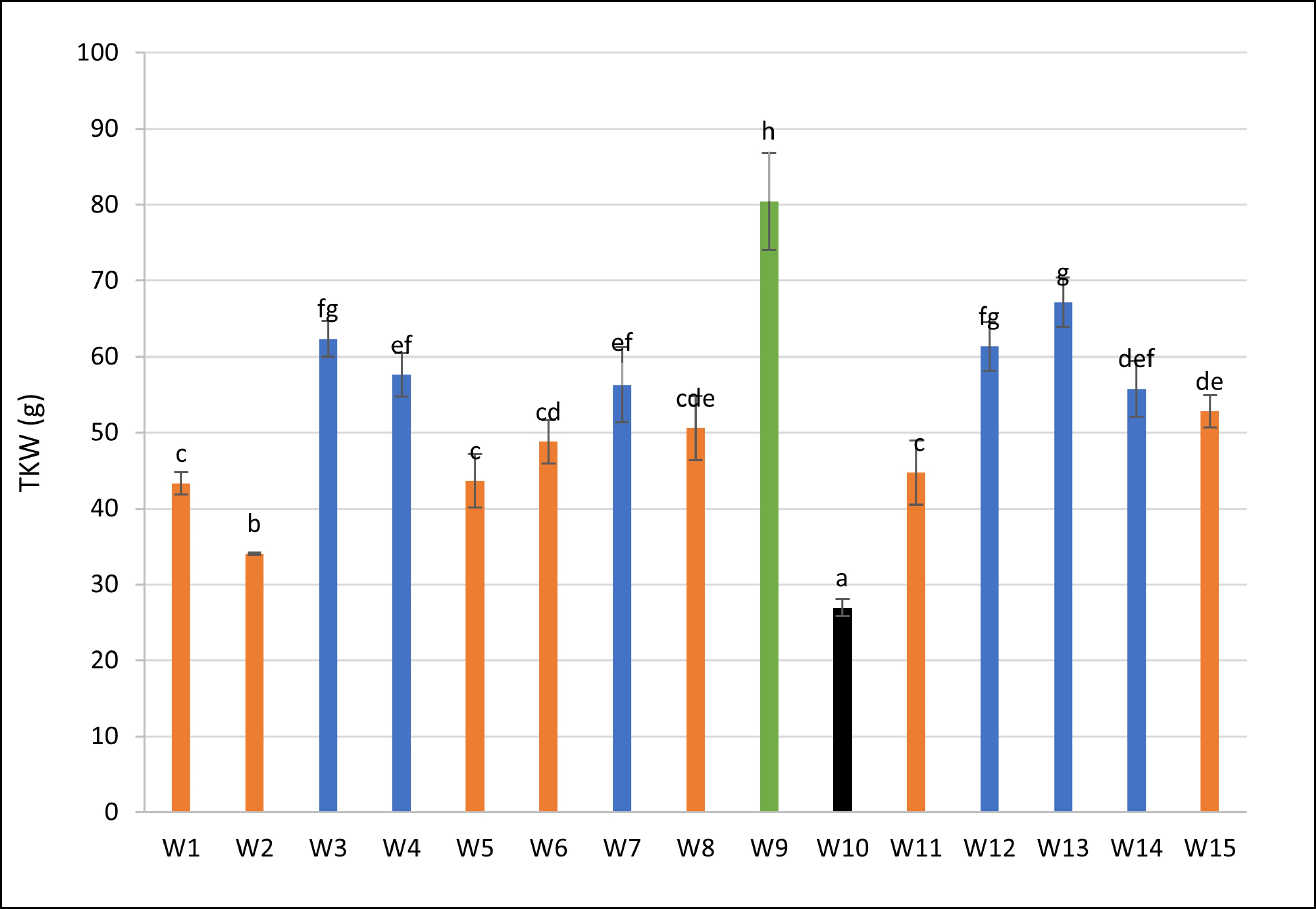
Due to their high heritability grain perimeter, area and volume are important indirect indices for grain yield improvement (Abdipour et al., 2016). Seed volume ranged from 48ml to 113ml (Elisavet - T. aestivum, cultivar, W2 and Leventis - T. polonicum, landrace, W9, respectively) (Table 4). It is worth noting that W9 (T. polonicum) had significantly the highest values of TKW, volume and seed firmness (SF). Although Papadakis (1929) mentioned that T. polonicum did not provide any agronomic value, our findings show that this species has bread-making value.
There are many methods to determine the required force to evaluate grain mechanical properties. In the present study, a rapid measurement of the mechanical properties of a single grain was used, which provided valuable results (Shewry & Hey, 2016). SF ranged from 62.96N to 194.85N (W10, Kopaida - T. dicoccum, landrace and W9, T. polonicum, landrace, respectively) and was correlated positively with seed weight and volume (0.840 and 0.791, P < 0.05, respectively) (Table 5). High values of weight and hardness indicate healthy seeds. Seed texture determines, to a great extent, milling properties and end uses. Softer seeds require more energy to mill and produce finer flours suitable for biscuit production, while flour from harder seeds is more appropriate for bread making (Liu, 2008). As W10 (Kopaida - T. dicoccum, landrace) had the lowest SF values, it could be suitable for cake and biscuit production. A recent research concluded that in general old cultivars of T. aestivum were softer than modern cultivars (Cetiner et al., 2020). In the present research, this statement was not fully confirmed, as there was no clear trend. However, it should be mentioned that fewer old and modern varieties were used in the present work compared to the earlier study.
Bulk density could be used as a criterion for yield improvement, as it was correlated positively with grain yield (Karimizadeh, Sharifi, & Mohammadi, 2012). In the present study, density did not differ significantly between accessions except for W10 (Kopaida - T. dicoccum, landrace), having the lowest value (0.55g/ml).
Seed flowability for all accessions was very good as indicated by Carr indices (CI) with values lower than 15% (Carr, 1965). W11 (Asprositi Kalavrita - T. aestivum, landrace) had the lowest CI value, 1.79%, and W13 (Mavragani Lemnos - T. durum, unknown status) had the highest value, 6.53%.
Accession code |
Seed firmness (N) |
Volume (ml) |
Tap Volume (ml) |
Bulk density (g/ml) |
Tap density (g/ml) |
CI (%) |
L* |
a* |
b* |
WI |
W1, Yekora |
88.82de |
61.50b |
60.50b |
0.70bc |
0.72bcde |
1.63a |
56.06de |
7.51ab |
24.47ab |
49.13cdef |
W2, Elisavet |
62.96a |
48.00a |
47.00a |
0.71bcd |
0.73bcde |
2.08a |
51.79bc |
10.21d |
23.78b |
45.26abc |
W3, Elpida |
101.00ef |
88.50h |
84.50g |
0.70bc |
0.74def |
4.52a |
58.00e |
6.80a |
23.98ab |
51.14f |
W4, Mexicali |
104.94fg |
81.50fg |
79.50f |
0.71bc |
0.72bcde |
2.45a |
53.61bcd |
7.61ab |
24.47b |
46.97bcde |
W5, Zoulitsa |
70.30ab |
65.00bc |
63.50bc |
0.67b |
0.69b |
2.31a |
50.97b |
9.05bcd |
21.34ab |
45.77abcd |
W6, Ntopio |
86.39cd |
68.50cd |
65.50c |
0.71bcd |
0.74ef |
4.38a |
50.03ab |
8.43bc |
21.37ab |
44.99ab |
W7, Mavragani Skyros |
105.43fg |
84.00gh |
80.50f |
0.67b |
0.70bc |
4.17a |
53.10bcd |
7.55ab |
24.29b |
46.64bcde |
W8, Grilos |
74.64abc |
74.00de |
72.00d |
0.68b |
0.70bcd |
2.70a |
51.76bc |
8.59bc |
22.86ab |
45.91abcd |
W9, Leventis |
194.85i |
113.00j |
110.00i |
0.70bc |
0.73cde |
4.35a |
49.42ab |
7.51ab |
21.35ab |
44.56ab |
W10, Kopaida |
59.29a |
49.00a |
47.00a |
0.55a |
0.57a |
4.08a |
46.14a |
8.07abc |
18.50a |
42.47a |
W11, Asprositi Kalavrita |
83.06bcd |
63.50bc |
63.00bc |
0.70bc |
0.71bcde |
0.79a |
57.12de |
7.77ab |
25.49b |
49.49def |
W12, Asprositi Kozani |
139.75h |
80.00efg |
78.33ef |
0.77d |
0.78g |
2.08a |
59.27e |
7.56ab |
25.40b |
51.40ef |
W13, Mavragani Lemnos |
93.09def |
99.50i |
93.00h |
0.67b |
0.72bcde |
6.53a |
55.68cde |
7.51ab |
24.97b |
48.55bcdef |
W14, Aspratheri |
117.94g |
75.00e |
72.00d |
0.74cd |
0.77fg |
4.00a |
53.93bcde |
9.97cd |
26.42b |
45.96abcde |
W15, Kokkinositaro |
83.07bcd |
77.00ef |
75.00de |
0.69b |
0.70bcd |
2.60a |
52.56bcd |
7.58ab |
22.31ab |
47.02abcdef |
In general, colour parameters did not differ significantly among accessions and were not correlated with the other physical properties (Table 5). The exceptions were the green/red hue (a*), which correlated negatively with volume (-0.516, P < 0.05), indicating that seeds were redder as their volume increased, and lightness (L*), blue/yellow hue (b*) and white index (WI), which were correlated positively with density (0.649, 0.719 and 0.565, P < 0.05, respectively). Increased density led to increased lightness, yellowness and whiteness of the seeds. W10 (Kopaida - T. dicoccum, landrace) was the only accession with a significantly lower value of b* among all other accessions. Colour determines to a great extent the overall consumer acceptance of food (Mandala & Protonotariou, 2021). Wheat kernel colour is related to different milling and baking attributes of the final products (Dowell, 1998). Bright yellow or amber colour of durum wheat results in high-quality pasta products (Cole, Johnson, Cole, & Stone, 1991), thus sample W10 (Kopaida - T. dicoccum, landrace) probably is the least suitable for pasta making among the studied accessions. Probably, W9 (Leventis - T. polonicum, landrace) and W13 (Mavragani Lemnos - T. durum, unknown status) could be the most suitable for pasta making considering their high values of b*, TKW and seed volume. However, further investigation is needed. Mechanical properties and sensory evaluation of produced pasta could verify our claim.
SF |
Volume |
Volume tap |
Bulk density |
Tap density |
TKW |
CI |
L* |
a* |
b* |
|
---|---|---|---|---|---|---|---|---|---|---|
Volume |
0.791 (0.000) |
|
|
|
|
|
|
|
|
|
Volume tap |
0.806 (0.000) |
0.998 (0.000) |
|
|
|
|
|
|
|
|
Bulk density |
0.462 |
0.283 (0.308) |
0.311 (0.259) |
|
|
|
|
|
|
|
Tap density |
0.500 |
0.401 (0.138) |
0.418 (0.121) |
0.969 (0.000) |
|
|
|
|
|
|
TKW |
0.840 (0.000) |
0.978 (0.000) |
0.982 (0.000) |
0.473 (0.075) |
0.574 (0.025) |
|
|
|
|
|
CI |
0.223 |
0.546 (0.035) |
0.496 (0.060) |
-0.271 (0.328) |
-0.053 (0.851) |
0.446 (0.096) |
|
|
|
|
L* |
0.170 |
0.214 (0.444) |
0.233 (0.403) |
0.649 (0.009) |
0.651 (0.009) |
0.337 (0.220) |
-0.215 (0.442) |
|
|
|
a* |
-0.317 |
-0.516 (0.049) |
-0.524 (0.045) |
0.100 (0.724) |
0.076 (0.787) |
-0.456 (0.088) |
-0.174 (0.535) |
-0.352 (0.198) |
|
|
b* |
0.197 |
0.192 (0.492) |
0.207 (0.460) |
0.719 (0.003) |
0.731 (0.002) |
0.326 (0.236) |
-0.166 (0.554) |
0.840 (0.000) |
0.014 (0.962) |
|
WI |
0.160 |
0.240 (0.389) |
0.260 (0.349) |
0.565 (0.028) |
0.565 (0.028) |
0.343 (0.210) |
-0.202 (0.472) |
0.974 (0.000) |
-0.501 (0.057) |
0.701 (0.004) |
The determination of kernel morphology is of great importance for bread making, as kernel shape and uniformity may influence the milling quality (Campbell et al., 1999). Image analysis could play a key role in distinguishing different wheat varieties by defining the product traceability for wheat landraces (Grillo, Blangiforti, & Venora, 2017; Khoshroo, Arefi, Masoumiasl, & Jowkar, 2014). In the present research, mean seed diameter ranged between 4.17mm (W2, Elisavet - T. aestivum, cultivar) and 5.66mm (W9, Leventis - T. polonicum, landrace). Particle size distribution curves differed between accessions, indicating that some landraces had a more uneven seed population than cultivars (Figure 6). Curve of W12 (Asprositi Kozani - T. durum, landrace) presented an almost bimodal distribution, indicating the presence of two distinguished populations of seeds, with different sizes, which could be explained by the fact that sometimes landraces consisted of two or more populations with different morphological characteristics (Papadakis, 1929). This could be linked with seed heteromorphism, characteristic of wild populations. A similar curve was presented by W13 (Mavragani Lemnos - T. durum, unknown status), where the bimodal distribution was less evident, but also presented a shoulder on the left (Figure 6). In contrast, W3 (Elpida - T. durum, cultivar) presented the narrowest unimodal distribution curve, with the peak around 5.5mm. All the other accessions presented frequency curves similar to W2 (Elisavet - T. aestivum, cultivar) or W9 (Leventis - T. polonicum, landrace). The main characteristic of these curves is that distribution is broad, due to the uneven seed populations. W10 (Kopaida - T. dicoccum, landrace) presented a similar curve to W2 (Elisavet - T. aestivum, cultivar), despite the fact that they belonged to different species.
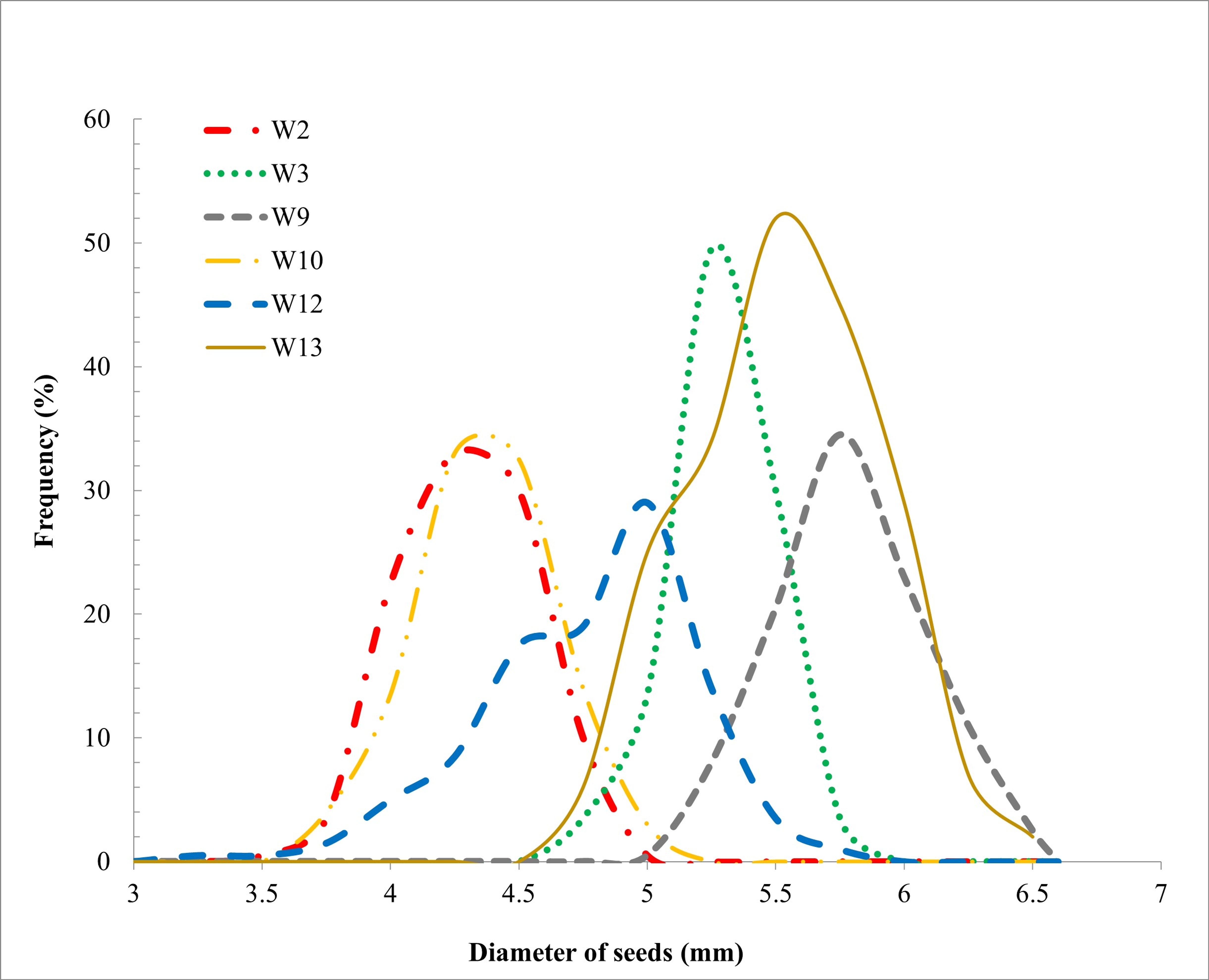
Bread wheat cultivars (W1, Yekora - T. aestivum and W2, Elisavet - T. aestivum) had significantly more roundness and shorter seeds compared to pasta wheat cultivars (W3, Elpida - T. durum, and W4, Mexicali 81 - T. durum), as referred to in the literature (Campbell et al., 1999). Perimeter was significantly lower for W2, Elisavet - T. aestivum, cultivar (15.71mm) and significantly higher for W9, Leventis - T. polonicum, landrace (23.62mm), compared to all other accessions (Supplemental Table 4). Similar results were observed for seed length, with W9 having the longest seeds (9.3mm) and W2 the shortest (5.96mm). T. polonicum (represented by W9) had characteristically long seed (Percival, 1921). Regarding the width of the seeds, the differences were not significant in all the cases and the values ranged from 2.72mm to 3.94mm (W10 and W13, respectively). Grain width could be used as a predictive index for mean grain weight determination (Haghshenas, Emam, & Jafarizadeh, 2022). However, in the present study, the most valuable parameter was the mean diameter.
The average grain area was 18.54mm2 (from 13.85mm2 to 26.08mm2, for W2, Elisavet - T. aestivum, cultivar and W9, Leventis - T. polonicum, respectively), in accordance with other studies (Abdipour et al., 2016; Gegas et al., 2010; Okamoto, Nguyen, Yoshioka, Iehisa, & Takumi, 2013). Only Kondić et al. (2020) recorded significantly higher values of seed area, 53.5mm2 and 40.17mm2 in two experimental years in Bosnia and Herzegovina for seeds of T. aestivum.
Correlations among all parameters
In the present study, TKW was significantly positively correlated with area of seed, mean diameter, SF, perimeter, length, width and volume of the seeds (0.871, 0.887, 0840, 0.786, 0.677, 0.829, and 0.975, P < 0.05, respectively, Supplemental Table 5). There is previous research which also associated kernel weight with its width and length (Campbell et al., 1999; Kondić et al., 2020), but some of them could not associate these parameters when they studied only bread wheats (Schuler, Bacon, Finney, & Gbur, 1995). Moreover, Javaid et al. (2005) found that TKW was positively correlated with plant height in Pakistani bread wheat landraces, and Moghaddam et al. (1997) found a significant correlation between TKW and number of tillers in Irani bread wheat landraces, which was not observed in the present study, suggesting possibly different patterns.
There are several studies evaluating wheat kernels by compression tests (Ponce-García, Ramírez-Wong, Escalante-Aburto, Torres-Chávez, & Figueroa-Cárdenas, 2016). However, there is a research gap on the correlation between wheat seed mechanical properties with plant-agromorphological and seed-morphological traits. In the present study, SF was significantly correlated with plant weight, and seed area, mean diameter, perimeter, length, width, volume and TKW (0.626, 0.711, 0.709, 0.688, 0.615, 0.570, and 0.840, P < 0.05, respectively). Thus, SF could be used as an additional tool to predict all the above parameters. Fitting a simple linear regression model to describe the relationship between SF and TKW explained 70.58% of firmness variability (P < 0.05) (Supplemental Figure 2).
Grouping of accessions
Cluster analysis, using all quantitative data, allowed us to group the wheat accessions in accordance with their species and breeding status (Figure 7). W9 (Leventis - T. polonicum, landrace) and W10 (Kopaida - T. dicoccum, landrace) created a distinct clade each, as anticipated, considering that they were different species. The control cultivars of T. durum species (W3 and W4) created a distinct cluster, along with the T. durum accessions of unknown breeding status W7 and W13 (Mavragani - T. durum, unknown status from Skyros and Lemnos, respectively). Interestingly, except for W11 (Asprositi Kalavrita) all landraces, whether T. aestivum or T. durum, formed a separate cluster, related to the T. aestivum cultivars.
It is worth noting that with the addition of physical properties in the cluster analysis, the grouping of Triticum spp. accessions changed compared to Figure 4, where only observations according to agromorphological traits were used. The cluster of landraces was closer to T. aestivum cultivars, indicating that landraces – regardless of the species they belonged to – could be more suitable for bread making, considering their physical properties, but also the main preference and use (mainly for bread) by farmers in Greece (Douma et al., 2016).
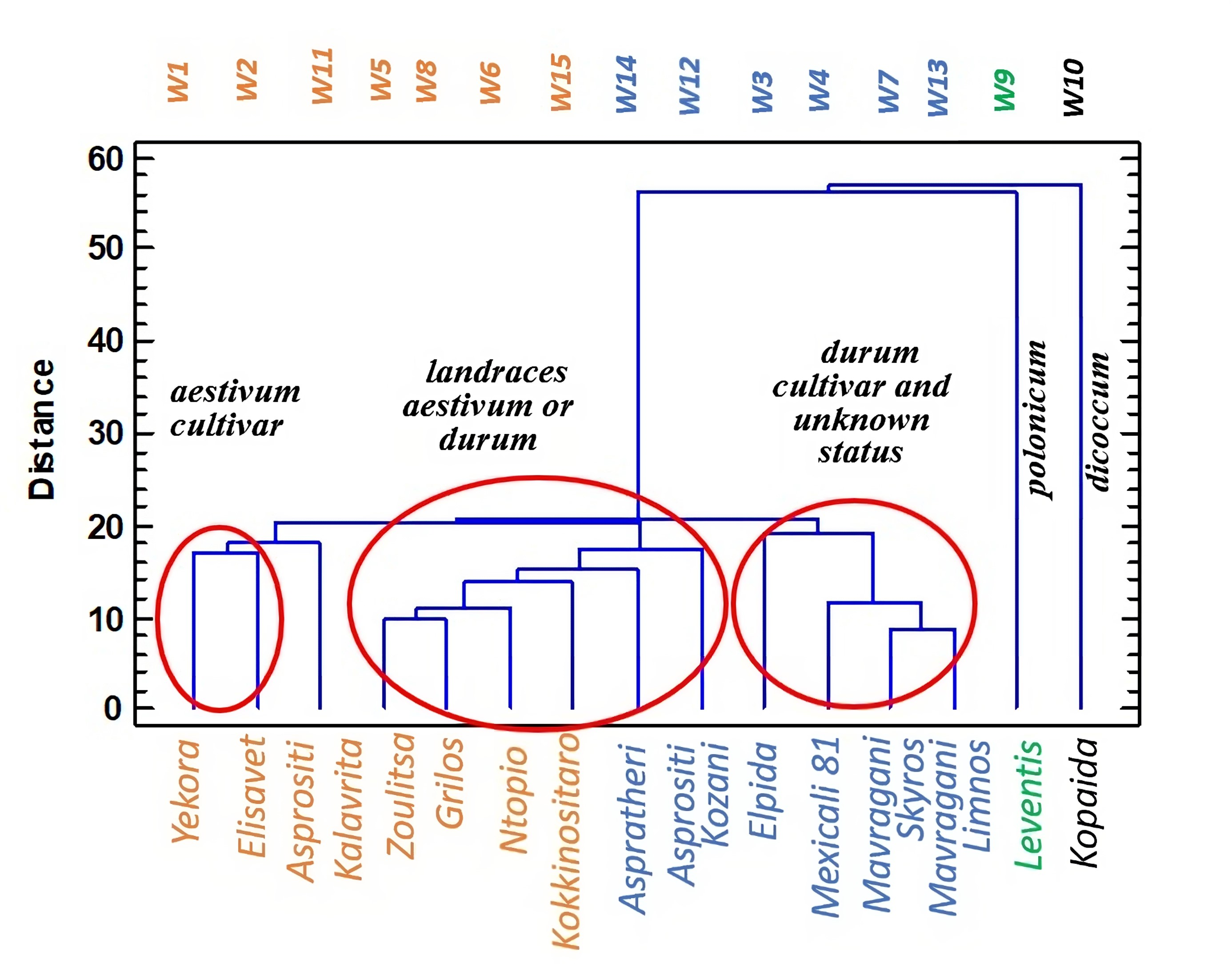
Conclusion
The agromorphological traits recorded in this study proved to be sufficient to highlight the differences between wheat species, landraces and cultivars, grouping them into two different clusters. TKW did not differ significantly between landraces and cultivars, indicating that landraces could also give a high flour yield. Increased values of TKW and seed volume indicated higher values of seed firmness. Cluster analysis of all data separated the wheat accessions into groups according to their attributes, indicating that Greek wheat landraces, either T. durum or T. aestivum could be probably used for bread making. Regarding the W10 (T. dicoccum, landrace) and W9 (T. polonicum, landrace) wheat accessions, their incorporation in both bread and pasta making would be of great interest, as they presented distinct characteristics. The evaluation of both agromorphological and physical properties of wheat seeds provided valuable information that could be used to distinguish wheat varieties for pasta and bread making based on quality characteristics.
Acknowledgements
The authors are grateful to Dr Michiel Van Slageren (Royal Botanical Gardens Kew, UK) who helped us with wheat taxonomy, to the student T. Sarros for helping with sowing, to the PhD candidates P. Chaloulos and P. Loukopoulos and Mr V. Protonotarios for helping with harvesting. Special acknowledgements go to the Institute of Plant Breeding and Genetic Resources and all the local producers for providing seeds of wheat cultivars and landraces, respectively. We thank the Hellenic National Meteorological Service for their prompt response to our request for meteorological data. We would also like to thank cordially the anonymous reviewers for their valuable comments and suggestions that improved the manuscript.
This research was co-financed by Greece and the European Union (European Social Fund-ESF) through the Operational Programme ‘Human Resources Development, Education and Lifelong Learning’ in the context of the project ‘Reinforcement of Postdoctoral Researchers – 2nd Cycle’ (MIS-5033021), implemented by the State Scholarships Foundation (ΙΚΥ).
Author contributions
SVP contributed to study conception and design, established the experimental trials, made field and laboratory measurements, performed data analysis and interpretation, and wrote the initial draft. RT contributed to study conception and design, conducted experiments on the agronomic research trial, provided the know-how in this field, worked on the draft and contributed to the writing of the final manuscript. AK contributed to statistical analysis, visualization and interpretation of the results. PJB contributed to the study conception and design, interpretation of the results, writing of the final manuscript, resource provision and supervision of the research regarding agronomic traits. IM contributed to study conception and design, interpretation of the results, final manuscript revision, resource provision and supervision of the research on the physical properties of seeds. All authors discussed the results and commented on the manuscript. All authors have read and agreed to the published version of the manuscript.
Conflict of interest
The authors declare no conflict of interest.
Supplemental data
Supplemental Figure 1: The frequency percentage for the four qualitative morphological traits observed in the 15 wheat accessions studied.
Supplemental Figure 2: Simple linear regression model to describe the relationship between seed firmness (SF) and thousand kernel weight (TKW) of wheat seeds.
Supplemental Table 1: Summary table of Friedman test results and medians of qualitative variables; plant growth habit; colour of ear; ear shape in profile and awn colour, for 15 Triticum spp accessions.
Supplemental Table 2: Analysis of Variance for quantitative agromorphological traits (ANOVA tables).
Supplemental Table 3: Spearman rank correlations between each pair of variables (qualitative: plant growth habit, colour of ear, ear shape in profile and awn colour versus all the variables).
Supplemental Table 4: Mean values of Image Analysis-Shape factor measurements of 15 Greek wheat accessions.
Supplemental Table 5: Pearson correlation test between quantitative parameters.